The Power of Sleep in Metabolic and Cardiovascular Health and the Profound Cost of its Deprivation
Key Points
- Sleep deprivation disrupts glucose metabolism and the typical secretion patterns of the appetite hormones, contributing to metabolic dysfunctions.
- Sleep hygiene is a valuable tool for cardiovascular disease prevention.
- Poor sleep quality shifts the autonomic nervous system balance towards sympathetic dominance, increasing the risk for cardiovascular disorders.
- The circadian rhythms of cortisol, adrenaline, and melatonin influence cardiovascular parameters, with disruptions due to sleep deprivation compromising cardiovascular health.
Our previous article about sleep discussed basic sleep concepts, such as sleep quality and duration, and their impact on recovery and exercise performance. In closing the chapter about sleep and its broad influences on overall health and well-being, the impact of sleep on metabolic and cardiovascular health will be analyzed in the second part. Metabolic health aspects, such as glucose regulation, appetite hormones, and energy balance, as well as cardiovascular health aspects, such as autonomic nervous system regulation and hormones, will be considered.
Sleep and metabolic health
Glucose regulation
In healthy individuals, glucose regulation appears to be under circadian control. Particularly, insulin sensitivity and glucose tolerance, which are major regulating factors of glucose metabolism, tend to be elevated during mornings and decrease as the day progresses. Circadian misalignment induced by sleep deprivation, poor sleep quality, or irregular sleeping patterns can disrupt these abilities, resulting in elevated blood glucose levels and extended time to restore normal levels. Thus, the chronically elevated blood sugar levels associated with systematic sleep deprivation can result in insulin resistance, leading to a high risk of developing a plethora of metabolic disorders, such as type II diabetes, obesity, and metabolic syndrome. Indicatively, robust data from experimental sleep restriction indicated that individuals with short sleep durations (≤6–7 h/night) are at 30% greater risk for developing type II diabetes.
Appetite hormones and energy balance
Another aspect of metabolism associated with circadian misalignment due to sleep disturbances is the 24-hour variation of the appetite hormones leptin and ghrelin. Leptin and ghrelin are primary coordinators of energy intake and energy expenditure throughout the day. Leptin is the satiety hormone that maintains energy balance by reducing caloric intake through decreased appetite, deterring fat production, and increasing energy expenditure. On the other hand, ghrelin is considered an orexigenic hormone, secreted mainly by the stomach. Ghrelin also plays a fundamental role in energy balance regulation with an opposite to leptin function.
Their functions are synchronized with leptin levels, which typically rise during the early stages of sleep and reach their lowest early in the morning, while ghrelin follows an inverse pattern. Ghrelin declines during bedtime and reaches its peak early in the morning, remaining high before meals and typically returning to baseline postprandially.
Although further research is required to elucidate the exact mechanisms, existing studies suggest that poor sleep quality and sleep deprivation interfere with appetite hormones’ secretion by altering the timing of their secretion, thus leading to dysregulation. Particularly, sleep restriction and irregular sleeping patterns are associated with diminished secretion of leptin and increased secretion of ghrelin, promoting elevated caloric intake and subsequent weight gain. From the evidence obtained so far, there might be a link between short sleep duration, adiposity, and the development of metabolic disorders.
The primary mechanism proposed for the dysregulation of appetite hormones due to sleep abnormalities is that sleep deprivation acts as a chronic stressor, affecting the hypothalamic-pituitary-adrenal axis function. The hypothalamic–pituitary–adrenal axis is responsible for cortisol production and secretion, an aforementioned stress hormone with catabolic properties. Sleep deprivation-induced stress can lead to elevated cortisol secretion by the brain, interfering with the normal signaling of the appetite hormones. As a consequence, leptin’s sensitivity is diminished, and even when the body has adequate energy reserves, the individual does not experience satiety. Additionally, cortisol can stimulate ghrelin secretion, further boosting feelings of hunger and potentially leading to weight gain.
Overall, sleep deprivation can have adverse effects on various aspects of metabolic health by disrupting glucose regulation, inducing stress, and potentially altering appetite control, resulting in a heightened risk of metabolic disorders like diabetes, type II diabetes, obesity, and metabolic syndrome.
Sleep and cardiovascular health
Autonomic Nervous System’s adaptations during healthy sleep
Adequate and quality sleep is considered fundamental in preserving cardiovascular health, as underlined in the recent guidelines released by the American College of Cardiology/American Heart Association. These guidelines state that counseling on sleep and its hygiene is a valuable approach to cardiovascular disease (CVD) prevention.
This importance can be attributed to the vital rest period sleep offers the cardiovascular system. Particularly, during sleep, cardiovascular regulation is modified, and many biological functions slow down, allowing the heart to rest from the stress of waking hours and facilitating efficient restoration of the cardiovascular tissues. In this context, the autonomic nervous system (ANS), which controls physiological body processes that occur despite our control, plays a central role. Specifically, a suppression of the sympathetic nervous system and increased parasympathetic system activity occurs. In other words, our body transitions from an alert state, often called the «fight or flight» response, to a state of relaxation and rejuvenation. This shift is predominantly evident during stages N1, N2, and N3 of NREM sleep.
During NREM sleep, heart rate and arterial blood pressure tend to decrease. This is because a shift towards a more parasympathetic tone occurs, promoting vasodilation (widening of blood vessels), enhancing blood flow to peripheral tissues, and thus fostering relaxation and tissue repair. On the contrary, during REM sleep, which involves the transition from sleep to wakefulness, our nervous system is predominantly under sympathetic control, leading to elevated heart rate and arterial blood pressure values.
The interplay between the sympathetic and parasympathetic activities can be efficiently reflected by HRV, a marker that serves as an indicator of the fluctuations in the time intervals between consecutive heartbeats. HRV normal values differ between populations and depend on factors like age, gender, and fitness level. During sleep, higher HRV values, meaning broader time variation between heartbeats, are associated with balanced autonomic nervous system activity and resilience to stress. Conversely, lower HRV indicates autonomic dysregulation, diminished adaptivity between states of alertness and relaxation, and potential susceptibility to cardiovascular issues.
Autonomic Nervous System’s adaptations during sleep deprivation
It’s becoming clear that the interplay between the two major branches of the autonomic nervous system (the sympathetic and parasympathetic nervous systems) is of great importance in achieving restful sleep. Disruptions of sleep duration and quality appear to disrupt this interplay, resulting in considerable predominance of the sympathetic nervous system’s activity. This suggests that sleep deprivation impairs the nervous system’s ability to adapt to shifting toward relaxation mode, subjecting individuals to a prolonged state of alertness and stress. As a result, a cascade of events occurs, including lower HRV values, increased proinflammatory cytokine secretion, and vasoconstriction, increasing the risk of hypertension, coronary artery disease, endothelial dysfunction, and other cardiovascular disorders.
Circadian Hormonal Dynamics and Cardiovascular Health
The circadian rhythm has implications for the cardiovascular system, as well. Hormones like cortisol, melatonin, and adrenaline, which follow circadian patterns, play a critical role in cardiovascular regulation.
As mentioned before, cortisol is a stress hormone, and its levels typically rise in the morning and reach their lowest during the night, enabling the anabolic hormones to facilitate recovery. However, chronic sleep deprivation can alter this hormonal synchronization, leading to dysregulated cortisol levels, potentially contributing to increased blood pressure and consequent risk of cardiovascular diseases.
Likewise, adrenaline, another hormone typically released as a response to stress stimuli, peaks throughout the day when the individual is active and the sympathetic nervous system governs. Sleep deprivation and irregular sleeping patterns can result in circadian misalignment associated with dysregulation of adrenaline secretion. This dysregulation leads to sympathetic nervous system dominance and, thus, elevated heart rate and blood pressure, meaning an increased risk for cardiovascular disorders.
Finally, melatonin, a hormone released by the brain, typically rises during the night as sleeping time approaches. Melatonin is known to exert cardioprotective effects through its antioxidant, anti-inflammatory, antiatherogenic, and lipid-lowering properties. Moreover, melatonin regulates blood pressure and vascular tone by contributing to blood pressure reduction during sleep. Hence, disruptions in melatonin secretion, often originating from chronic insufficient or poor sleep, pose a non-negligent risk for cardiovascular diseases.
To sum up, adequate sleep quality is a good indicator of metabolic and cardiovascular health. Therefore, prioritizing sufficient and quality sleep for the optimal functioning of the cardiovascular and metabolic health system and thus for longevity is crucial and should not be ignored since sleep is, along with nutrition and exercise, amongst the main pillars of a healthy, long life.
REFERENCES
Baranwal N, Yu PK, Siegel NS. Sleep physiology, pathophysiology, and sleep hygiene. Prog Cardiovasc Dis. 2023 Mar-Apr;77:59-69. DOI:
https://doi.org/10.1016/j.pcad.2023.02.005
Besedovsky L, Lange T, Born J. Sleep and immune function. Pflugers Arch. 2012 Jan;463(1):121-37. DOI:
10.1007/s00424-011-1044-0
Huang W, Ramsey KM, Marcheva B, Bass J. Circadian rhythms, sleep, and metabolism. J Clin Invest. 2011 Jun;121(6):2133-41. DOI:
10.1172/JCI46043
Korostovtseva L, Bochkarev M, Sviryaev Y. Sleep and Cardiovascular Risk. Sleep Med Clin. 2021 Sep;16(3):485-497. DOI:
https://doi.org/10.1016/j.jsmc.2021.05.001
Lin J, Jiang Y, Wang G, Meng M, Zhu Q, Mei H, Liu S, Jiang F. Associations of short sleep duration with appetite-regulating hormones and adipokines: A systematic review and meta-analysis. Obes Rev. 2020 Nov;21(11):e13051. DOI:
https://doi.org/10.1111/obr.13051
Liu S, Wang X, Zheng Q, Gao L, Sun Q. Sleep Deprivation and Central Appetite Regulation. Nutrients. 2022 Dec 7;14(24):5196. DOI:
https://doi.org/10.3390/nu14245196
Morselli L, Leproult R, Balbo M, Spiegel K. Role of sleep duration in the regulation of glucose metabolism and appetite. Best Pract Res Clin Endocrinol Metab. 2010 Oct;24(5):687-702. DOI:
https://doi.org/10.1016/j.beem.2010.07.005
Rogers EM, Banks NF, Jenkins NDM. The effects of sleep disruption on metabolism, hunger, and satiety, and the influence of psychosocial stress and exercise: A narrative review. Diabetes Metab Res Rev. 2024 Feb;40(2):e3667. DOI:
https://doi.org/10.1002/dmrr.3667
Tobaldini E, Costantino G, Solbiati M, Cogliati C, Kara T, Nobili L, Montano N. Sleep, sleep deprivation, autonomic nervous system and cardiovascular diseases. Neurosci Biobehav Rev. 2017 Mar;74(Pt B):321-329. DOI:
https://doi.org/10.1016/j.neubiorev.2016.07.004
Troynikov O, Watson CG, Nawaz N. Sleep environments and sleep physiology: A review. J Therm Biol. 2018 Dec;78:192-203. DOI:
https://doi.org/10.1016/j.jtherbio.2018.09.012
An Ounce of Prevention - Hyperion Health Blog
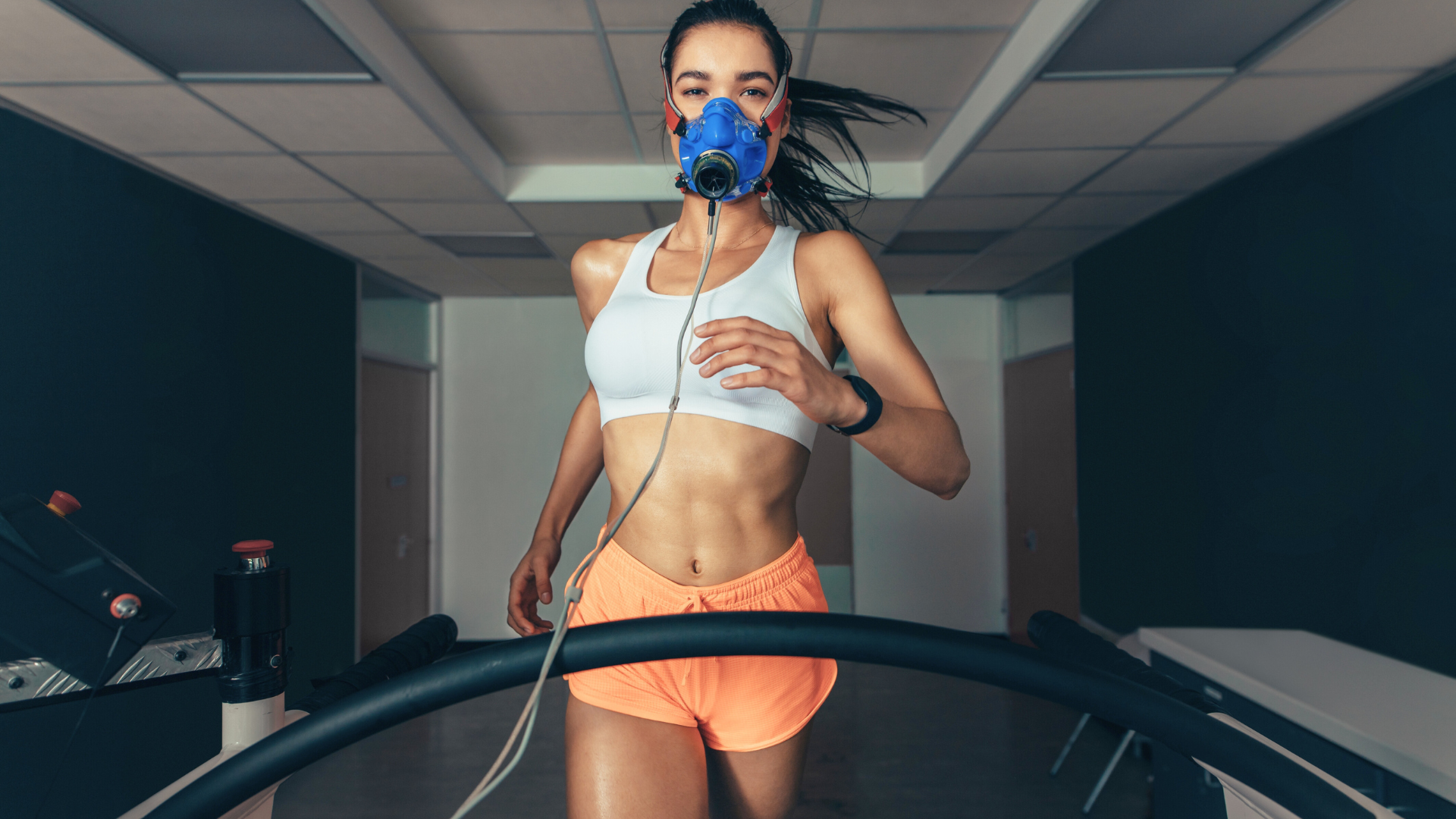
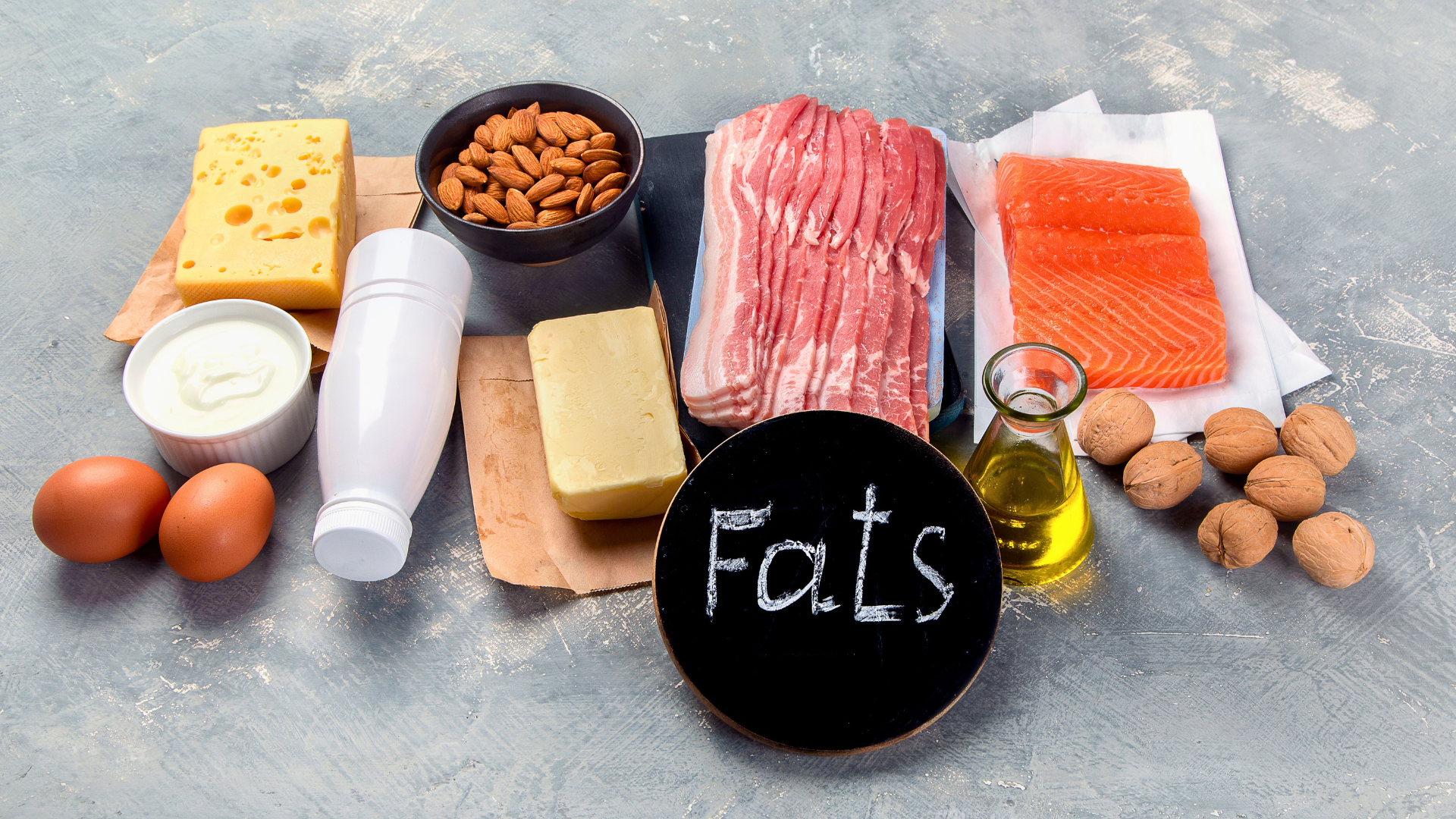