An Ounce of Prevention:
Hyperion Health Blog
An Ounce of Prevention - Hyperion Health Blog
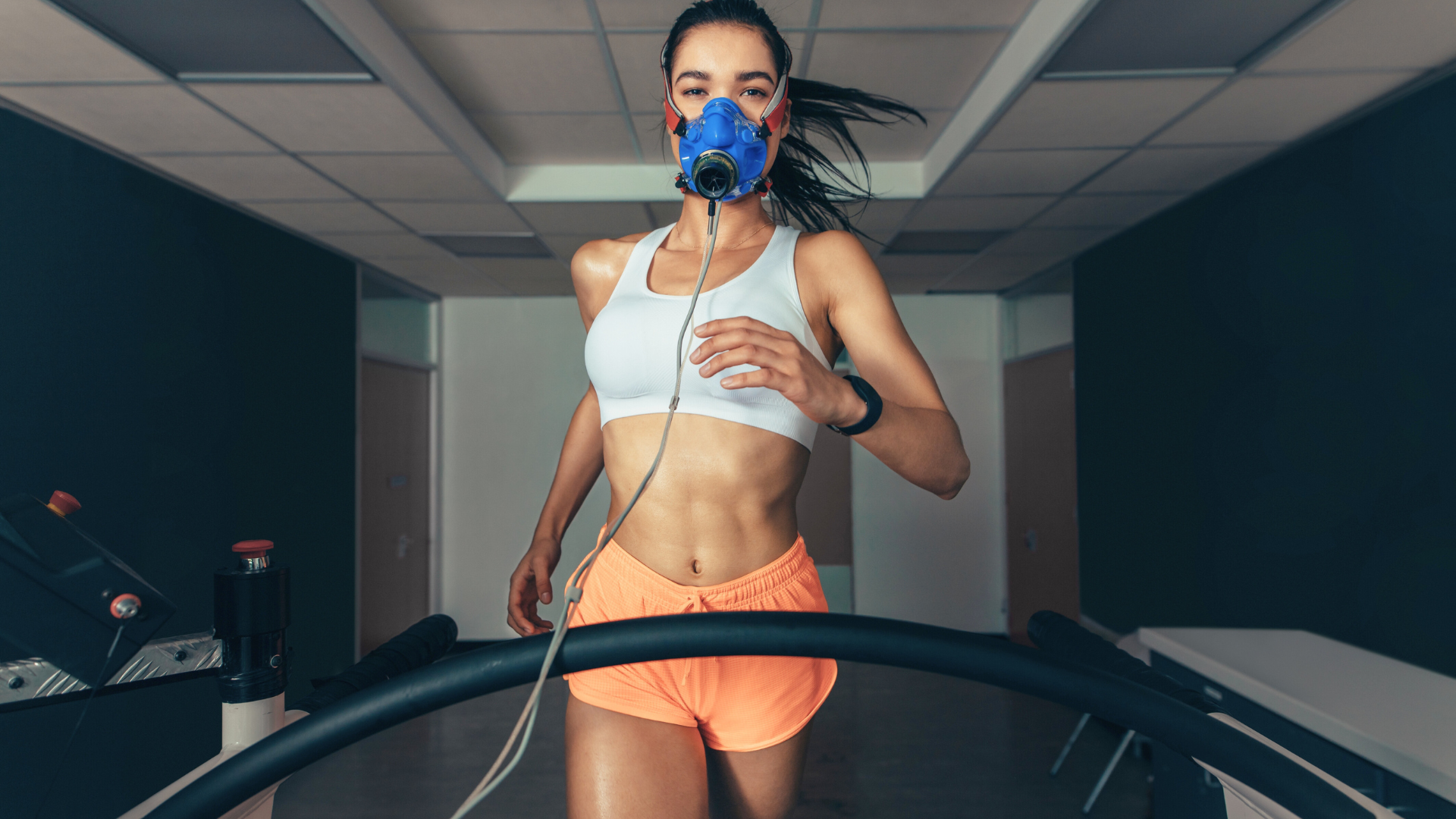
Highlights Healthcare expenses are skyrocketing, with consumers and employers facing the significant brunt. Identifying those likely to get sick is critical as our resource-strapped healthcare system should focus on those likely to become the most significant burden to the system. VO2 max is a crucial longevity indicator that can also accurately predict healthcare expenses. The rampant chronic disease epidemic and the resulting surge in medical expenses is one of the most dire problems of modern societies, probably only second to climate change. Healthcare inflation is on a meteoric rise, and for those with limited or no healthcare coverage, a medical emergency is the equivalent of personal bankruptcy. A dire problem for employers In the US, employers and consumers who face rising health insurance premiums and astronomical out-of-pocket medical expenses feel the brunt of rising healthcare costs. Such is the problem that even large, well-capitalized corporations choose to send employees overseas for specific medical procedures since the cost of traveling and treatment in a foreign country is lower than the cost of care in the US. Another startling example is the infamous "northern caravan," a term that describes people with diabetes in the northern states who travel to Canada to secure their insulin supply. According to McKinsey , a survey conducted among over 300 employers highlighted that the average increase in the cost of health benefits over the past three years has been within the range of 6 to 7 percent. This survey also indicated that any rate increases exceeding 4 to 5 percent were deemed unsustainable. Interestingly, 95 percent of the surveyed employers expressed willingness to contemplate reducing benefits if costs surged by 4 percent or more. The primary cost-control measures that these employers indicated they might explore included elevating the portion of premium costs covered by employees and a potential transition to high-deductible health plans. Why is Breath Analysis relevant? Vis-a-vis this problem, the early and accurate estimation of who will get sick and how much they will cost is as critical as the treatment itself. The reason is that no other method of accurately identifying at-risk populations exists; it helps focus our scarce prevention resources and attention on those most in need. Breath analysis, AKA VO2max or metabolic testing, is an assessment that reveals two key biomarkers that provide significant predictive value for one's likelihood of developing costly chronic conditions. These two biomarkers are VO2max and the Respiratory Exchange Ratio. In this article, we will dive into VO2max to understand why it's a critical reflection of our overall health and, consequently, a window into our future healthcare spend. What is VO2max? Let's start with the basics. What is VO2max? VO2 max is the maximum amount of oxygen the human body can absorb. It is measured in terms of milliliters of oxygen consumed per kilogram of body weight. The below formula below indicates how VO2max is calculated: The numerator indicates the volume of oxygen your heart, lungs, and cells can absorb, expressed in milliliters per minute. The denominator indicates the weight of the individual represented in kilograms.
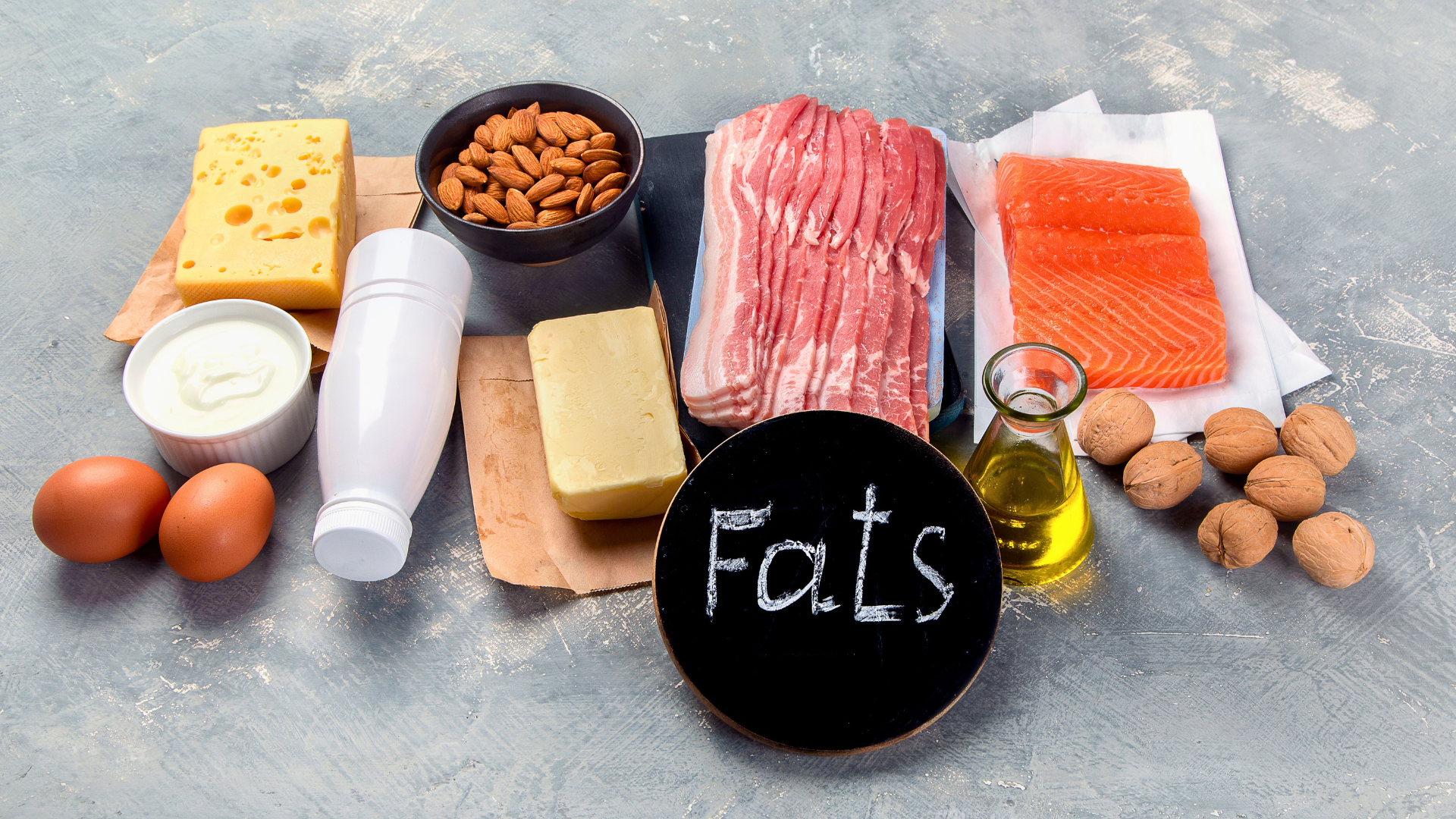
Key points A total fat intake between 20-35% ensures sufficient intake of essential fatty acids and fat-soluble vitamins Omega-6 PUFAs are primarily found in vegetable oils, while omega-3 PUFAs are primarily found in fatty fish and fish oils Both omega-3 PUFAs and MUFAs have established benefits for cardiovascular disease TFAs are the only dietary lipids that have a strong positive relationship with cardiovascular disease Omega-3 PUFA supplementation increases the beneficial bacteria of the human microbiome Over the last three decades, there has been a great revolution against fat due to its suspected association with several nutritional health issues, especially cardiovascular disease. There was a tremendous amount of evidence that indicated dietary cholesterol and saturated fat as the main culprits of cardiovascular disease, thus morbidity and mortality. It was when all the low-fat and no-fat dairy products started to launch, promising even complete substitution of the cholesterol-lowering heart medication if these products were exclusively consumed. Let’s start from the beginning. Dietary fat intake can vary significantly and still meet energy and nutrient needs. International guidelines suggest a total fat intake between 20% and 35% of the daily caloric consumption. This range ensures sufficient intake of essential fatty acids and fat-soluble vitamins. Not only does the quantity of the ingested fat matter, but most importantly, its quality. Some dietary fats have beneficial effects, with a significant role in maintaining good health, while others may threaten it. Which are, after all, the dietary fats? Dietary fats is a rather heterogeneous group of organic compounds, including four main types of fat, which are elaborately described in the following sections of this article. Polyunsaturated fatty acids (PUFAs) Polyunsaturated fatty acids (PUFAs) have two or more carbon-carbon double bonds. Omega-6 PUFAs and omega-3 PUFAs are the main types of PUFAs and are classified according to the location of the first unsaturated bond (sixth and third carbon atom, respectively). Alpha-Linolenic acid (ALA), docosahexaenoic acid (DHA), docosapentaenoic acid (DPA), and eicosapentaenoic acid (EPA) are the most important omega-3 PUFAs. ALA is an essential fatty acid that can only be obtained from diet and can be converted into EPA and then to DHA, but the rate of this conversion is finite, approximately 7.0%–21% for EPA and 0.01%–1% for DHA. In the same way, the most important omega-6 PUFAs are linoleic acid (LA) and arachidonic acid (ARA). LA is an essential fatty acid that, in order to give rise to ARA, needs to be ingested through the diet as the human body cannot synthesize it. The recommended intake for total PUFA ranges between 5% and 10% of the total energy intake, while a total omega-3 PUFA intake of 0.5%–2% and a total omega-6 PUFA intake of 2.5%-5% is suggested. A dietary ratio of omega−6/omega−3 PUFA is recommended to be 1:1–2:1 to balance their competing roles and achieve health benefits. Omega-6 and omega-3 PUFAs Omega-6 PUFAs, in the form of LA, are plentiful in most crop seeds and vegetable oils, such as canola, soybean, corn, and sunflower oils. In contrast to omega-6 PUFAs, omega-3 PUFAs are obtained from a limited range of dietary sources. Flax, chia, and perilla seeds are rich in ALA, with significant amounts also detected in green leafy vegetables. The consumption of fatty fish, such as salmon, sardines, tuna, trout, and herring, provides high amounts of EPA and DHA. Besides fish and their oils, small amounts of omega-3 PUFAs are also detected in red meat like beef, lamb, and mutton. All the above dietary sources provide EPA, DPA, DHA, LA, and ARA in different amounts, and their intake is necessary for normal physiological function. PUFAs play a critical role in many chronic diseases, affecting human cells by regulating inflammation, immune response, and angiogenesis. Omega-3 PUFAs’ role against hypertriglyceridemia has been clarified, and research indicates that systematically consuming oily fish can contribute to general heart protection. Supplementation with omega-3 PUFAs could potentially lower the risk of several cardiovascular outcomes, but the evidence is stronger for individuals with established coronary heart disease. Moreover, adequate EPA and DHA levels are necessary for brain anatomy, metabolism, and function. Although the mechanisms underlying omega-3 PUFAs' cardioprotective effects are still poorly understood, several studies have been conducted in this direction. Unfortunately, that does not hold true for their omega-6 counterparts, for which controversial emerging data tend to show anti-inflammatory behavior that needs to be further studied. Monounsaturated fatty acids (MUFAs) In contrast to PUFAs, monounsaturated fatty acids (MUFAs) are easily produced by the liver in response to the ingestion of carbohydrates. The main MUFA is oleic acid, found in plant sources, such as olive oil, olives, avocado, nuts, and seeds, while minimal amounts are also present in meat, eggs, and dairy products. Specific guidelines around MUFAs’ dietary consumption do not exist. Therefore, MUFAs are recommended to cover the remaining fat intake requirements to reach the total daily fat intake goal. A growing body of research shows that dietary MUFAs reduce or prevent the risk of metabolic syndrome, cardiovascular disease (CVD), and hypertension by positively affecting insulin sensitivity, blood lipid levels, and blood pressure, respectively. Moreover, olive oil contains several bioactive substances, possessing anti-tumor, anti-inflammatory, and antioxidant qualities. According to a meta-analysis, consuming olive oil was linked to a lower risk of developing any sort of cancer, especially breast cancer and cancer of the digestive system. Another study found that an isocaloric replacement of 5% of the energy from saturated fatty acids (SFAs) with plant MUFAs led to an 11% drop in cancer mortality over a 16-year follow-up period. Therefore, including MUFAs in the everyday diet offers multifaceted benefits in chronic disease prevention and management, including cancer and general health promotion. Saturated fatty acids (SFAs) Saturated fatty acids (SFAs) form a heterogeneous group of fatty acids that contain only carbon-to-carbon single bonds. Whole-fat dairy, (unprocessed) red meat, milk chocolate, coconut, and palm kernel oil are all SFA-rich foods. These fatty acids have distinct physical and chemical profiles and varying effects on serum lipids and lipoproteins. Stearic, palmitic, myristic, and lauric acids are the principal SFAs found in most natural human diets. Dietary practice and guidelines recommend limiting SFA intake to <10% of the total energy (E%), while the American Heart Association suggests an even lower intake of <7 E% because total saturated fat consumption and LDL-C levels are positively correlated. However, the role of SFAs in CVDs is quite complex, and the evidence is heterogeneous. In a recent study with a 10.6-year follow-up period, which included 195,658 participants, there was no proof that consuming SFAs was linked to developing CVD while replacing saturated fat with polyunsaturated fat was linked to an increased risk of CVD. Moreover, according to 6 systematic reviews and meta-analyses, cardiovascular outcomes and total mortality were not significantly impacted by substituting saturated fat with polyunsaturated fat. Even if these analyses were to be challenged, due to heterogenous evidence, the possible reduction in CVD risk associated with replacing SFAs with PUFAs in several studies may not necessarily be an outcome of SFAs’ negative effect but rather a potential positive benefit of PUFAs. Regarding SFAs' effect on different types of cancers, associations of their intake with an increased risk of prostate and breast cancer have been indicated. Conversely, a meta-analysis showed no link between SFA intake and a higher risk of colon cancer; similarly, consuming MUFAs, PUFAs, or total fat did not affect colon cancer risk. Hence, the role of SFA consumption in preventing, promoting, or having a neutral role in serious chronic diseases has not been fully elucidated yet. Trans fatty acids (TFAs) Trans fatty acids (TFAs) are created industrially by partially hydrogenating liquid plant oils or can be naturally derived from ruminant-based meat and dairy products. TFAs are highly found in commercial baked goods, biscuits, cakes, fried foods, etc. Guidelines regarding TFAs are stringent and limit TFA intake to <1% of energy or as low as possible. In 2015, the US Food and Drug Administration declared that industrial TFAs are no longer generally recognized as safe and should be eliminated from the food supply as their consumption is strongly linked to various CVD risk factors. Specifically, TFA intake raises triglycerides and increases inflammation, endothelial dysfunction, and hepatic fat synthesis, leading to a significantly increased risk of coronary heart disease (CHD). A meta-analysis suggested that increased TFA intake led to an increase in total and LDL-cholesterol and a decrease in HDL-cholesterol concentrations. Data also indicates that TFAs may influence carcinogenesis through inflammatory pathways, but the reported data are debatable. A recent study investigated the effects of all types of dietary fat intake on CVD risk. While PUFA, MUFA, and SFA intake were not linked to higher CVD risk, dietary TFA intake showed a strong association with CVD risk. Analysis indicated PUFA intake and CVD risk were inversely correlated, and the relative risk of CVD was reduced by 5% in studies with a 10-year follow-up. Dietary lipids and the human microbiome Dietary lipids also affect human microbiota composition. Studies have identified a close association between the human microbiome and metabolic diseases, including obesity and type 2 diabetes. Diets with a high omega-6 PUFA, SFA, and TFA intake increase the amount of many detrimental bacteria in the microbiome and reduce the amount of the beneficial ones, altering the microbiota composition and inducing inflammation via the secretion of pro-inflammatory cytokines. These bacteria may disrupt the gut barrier function, allowing lipopolysaccharides (LPS) translocation, which are bacterial toxins. This condition is linked to metabolic perturbations such as dyslipidemia, insulin resistance, non-alcoholic fatty liver disease (NAFLD), and CVD. On the contrary, omega-3 PUFA (EPA and DHA) supplementation increases beneficial bacteria and limits harmful ones, enhancing intestinal barrier functioning and preventing LPS translocation and its implications. Omega-3 PUFA supplementation has also been studied as a means of mental health disorders management, but the evidence is still controversial. A possible protective impact of fish consumption on depression has been suggested by various studies, as well as a possible protective effect of dietary PUFAs on moderate cognitive impairment. A recent review of meta-analyses indicated that omega-3 PUFA supplementation might have potential value in mental health disorders, but data credibility is still weak. Dietary lipids and obesity Last but not least, obesity and its management is another field that dietary lipids intake seems to impact with their mechanisms. A diet high in PUFA has been shown to lower the total mass of subcutaneous white adipose tissue (the predominant fat type in human bodies), reduce blood lipid levels, and improve insulin sensitivity. In a study comparing PUFA and MUFA isocaloric intake, PUFA was more advantageous and lowered visceral adiposity in patients with central obesity. By stimulating brown adipose tissue, which aids energy expenditure through its elevated thermogenic activity, omega-3 PUFAs seem to elicit these positive effects in fat tissue, thus being useful in preventing and/or managing obesity. Another related study compared PUFA to SFA overfeeding in dietary surplus conditions that aimed to increase weight by 3%. While SFA overfeeding led to weight gain, primarily through the expansion of the visceral adipose tissue, PUFA overfeeding also led to weight gain, but because of a greater expansion of lean tissue mass. To sum up, dietary fats are an essential part of the human diet with many important physiologic functions, including cell function, hormone production, energy, and nutrient absorption. Moreover, dietary fat consumption is associated with positive outcomes in regard to cardiovascular disease, metabolic syndrome, cancer, and depression. Therefore, there is no reason to demonize this valuable dietary component, incriminating it for irrelevant adverse health outcomes, primarily weight loss failure and obesity. References 1. Astrup A, Magkos F, Bier DM, Brenna JT, de Oliveira Otto MC, Hill JO, King JC, Mente A, Ordovas JM, Volek JS, Yusuf S, Krauss RM. Saturated fats and health: A reassessment and proposal for food-based recommendations: JACC State-of-the-Art review. J Am Coll Cardiol. 2020;76(7):844-857. DOI: 10.1016/j.jacc.2020.05.077 2. Bojková B, Winklewski PJ, Wszedybyl-Winlewska M. Dietary fat and cancer-Which is good, which is bad, and the body of evidence. Int J Mol Sci. 2020;21(11):4114. DOI: 10.3390/ijms21114114 3. Custers, Emma EM, Kiliaan, Amanda J. Dietary lipids from body to brain. Prog Lipid Res. 2022;85:101144. DOI: 10.1016/j.plipres.2021.101144 4. de Souza RJ, Mente A, Maroleanu A, Cozma AI, Ha V, Kishibe T, Uleryk E, Budylowski P, Schünemann H, Beyene J, Anand SS. Intake of saturated and trans unsaturated fatty acids and risk of all cause mortality, cardiovascular disease, and type 2 diabetes: systematic review and meta-analysis of observational studies. BMJ. 2015;351:h3978. DOI: 10.1136/bmj.h3978 5. Gao X, Su X, Han X, Wen X, Cheng C, Zhang S, Li W, Cai J, Zheng L, Ma J, Liao M, Ni W, Liu T, Liu D, Ma W, Han S, Zhu S, Ye Y, Zeng F-F. Unsaturated fatty acids in mental disorders: An umbrella review of meta-analyses. Adv Nutr. 2022;13(6):2217-2236. DOI: 10.1093/advances/nmac084 6. Liu AG, Ford NA, Hu FB, Zelman KM, Mozaffarian D, Kris-Etherton PM. A healthy approach to dietary fats: understanding the science and taking action to reduce consumer confusion. Nutr J. 2017;16(1):53. DOI: 10.1186/s12937-017-0271-4 7. Poli A, Agostoni C, Visioli F. Dietary fatty acids and inflammation: Focus on the n-6 series. Int J Mol Sci. 2023;24(5):4567. DOI: 10.3390/ijms24054567 8. Saini RK, Keum Y-S. Omega-3 and omega-6 polyunsaturated fatty acids: Dietary sources, metabolism, and significance-A review. Life Sci. 2018;203:255-267. DOI: 10.1016/j.lfs.2018.04.049 9. Saini RK, Prasad P, Sreedhar RV, Naidu KA, Shang X, Keum Y-S. Omega-3 polyunsaturated fatty acids (PUFAS): Emerging plant and microbial sources, oxidative stability, bioavailability, and health benefits-A review. Antioxidants (Basel). 2021;10(10):1627. DOI: 10.3390/antiox10101627 10. Zhao M, Chiriboga D, Olendzki B, Xie B, Li Y, McGonigal LJ, Maldonado-Contreras A, Ma Y. Substantial increase in compliance with saturated fatty acid intake recommendations after one year following the American Heart Association diet. Nutrients. 2018;10(10):1486. DOI: 10.3390/nu10101486 11. Zhu Y, Bo Y, Liu Y. Dietary total fat, fatty acids intake, and risk of cardiovascular disease: a dose-response meta-analysis of cohort studies. Lipids Health Dis. 2019;18:91. DOI: 10.1186/s12944-019-1035-2
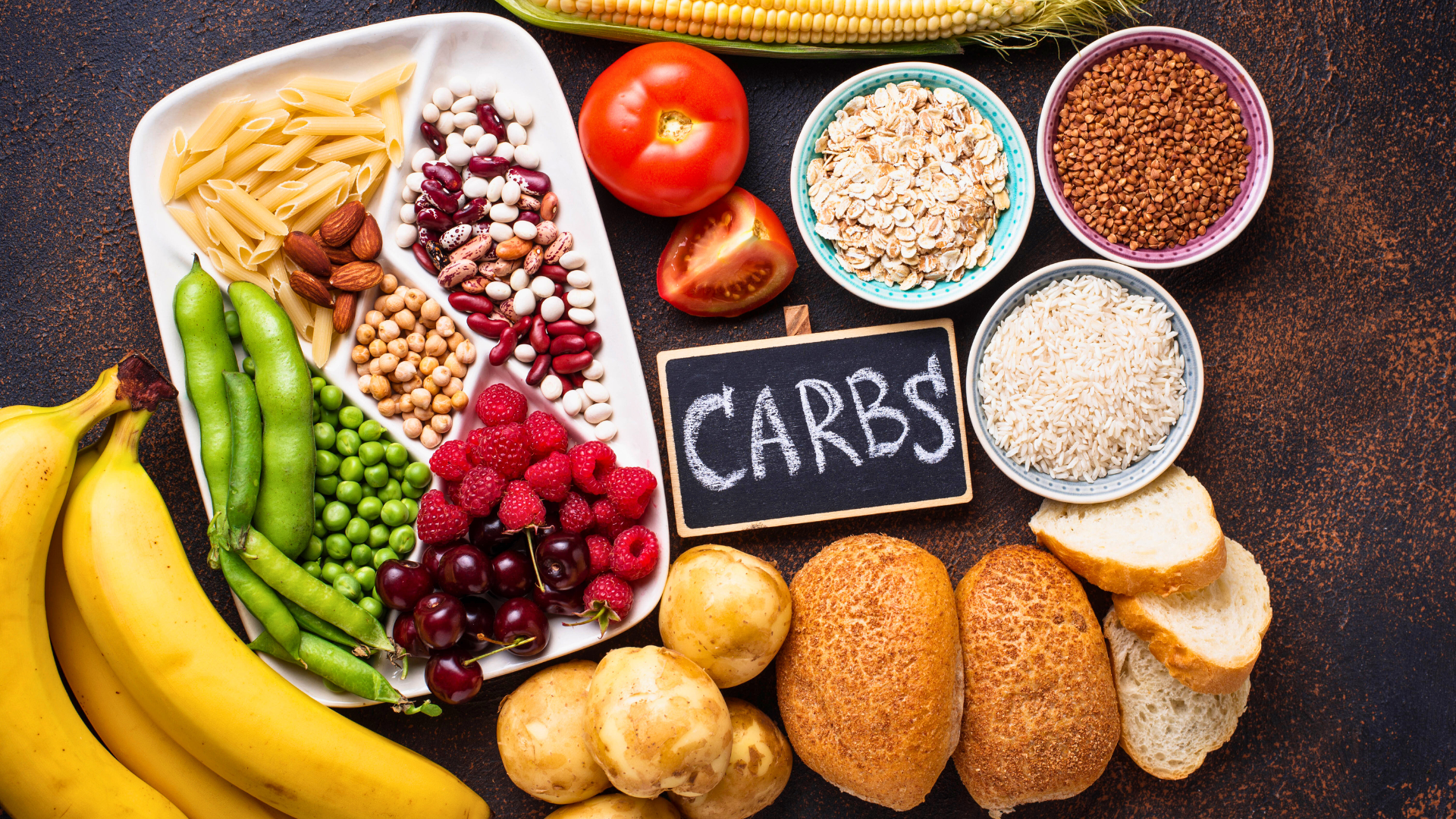
Key points A recommended total carbohydrate intake ranges between 45-60% of the daily calories with a bottom value of 130 g/day to ensure the maintenance of adequate brain function Metabolic disorders, such as obesity and diabetes, disrupt fat utilization at rest, promoting an increased reliance on carbohydrates for energy production The phosphagen energy system, aerobic oxidative system, and anaerobic lactic system are the three energy systems activated during different types of exercise Consuming a rich in complex carbohydrates meal 2-3 hours before engaging in endurance exercise lasting >60 minutes can help prevent hypoglycemia Optimal post-workout recovery is achieved with co-ingestion of 1-2.2 g/kg carbohydrates and 0.3-5 g/kg proteins in a post-workout meal Excessive consumption of simple carbohydrates found in processed foods, refined grains, and deserts poses considerable risks for metabolic and overall health Complex carbohydrates, especially dietary fibers, found in whole grains, fruits, and vegetables, offer diverse health advantages Over the years, social media has fostered a negative view towards carbohydrate (CHO) consumption, advocating that dietary patterns aiming at weight loss should limit or even exclude carbohydrates. These beliefs intensify the debate about the dietary intake of the three primary energy sources (carbohydrates, proteins, and fats) during rest and physical activity. Individuals derive about half of their daily energy requirements from carbohydrates on average. However, food abundance and easy accessibility have led to excess carbohydrate intake, particularly plain sugars, placing a significant metabolic burden on the body. Therefore, being mindful of carbohydrates' quantity, quality, and distribution throughout the day is essential for establishing a well-balanced approach to nutrition and maintaining overall health. Carbohydrate classification Carbohydrates are the primary energy source of the human diet. Particularly, each gram of any type of CHO renders four calories. They can be classified into simple and complex carbohydrates, depending on the amount of sugar they contain. Simple carbohydrates Simple carbohydrates are short-chain sugar molecules that can be quickly digested, inducing a sharp increase in blood glucose levels. This feature makes them an immediate source of energy. However, the blood glucose spikes are followed by an equally rapid drop in glucose levels, leading to feelings of hunger and fatigue. This type of carbohydrate is divided into two major categories, monosaccharides, and disaccharides, based on the sugar units they consist of. Monosaccharides are composed of a single sugar unit and include glucose, fructose, and galactose. They are naturally present in honey and dried fruits but can also be found in elevated quantities in manufactured goods. Fructose, for example, particularly high-fructose corn syrup, is a constituent of many soft drinks and processed foods. Disaccharides, on the other hand, consist of two sugar units, composing sucrose, lactose, and maltose. Sucrose is naturally derived from sugarcane, sugar beets, honey, and dates, while lactose is the sugar of dairy products. Maltose is predominantly found in beer, barley, and various cereals. Complex carbohydrates Complex carbohydrates are also divided into subgroups: oligosaccharides, polysaccharides, and dietary fiber. Oligosaccharide molecules constitute a chain of 3-10 sugar units, while polysaccharides comprise ≥ 10 sugar units. Fibers are a distinct category, including both oligo- and polysaccharide components. Contrary to simple carbohydrates, complex carbohydrates increase blood glucose progressively, providing prolonged feelings of satiety. As their digestion and absorption are gradual, quick energy depletion is averted. Raffinose, stachyose, maltodextrin, and inulin are well-known representatives of the group of oligosaccharides. Various fruits, vegetables, legumes, and whole grains are rich in oligosaccharides. Likewise, typical polysaccharides encompass glycogen and starch. Polysaccharides exist in high concentrations in plant-based food sources such as fruits, vegetables, legumes, whole grains, and nuts. Several oligo- and polysaccharides belong to another special type of complex carbohydrates: dietary fiber. Examples of dietary fibers are pectins, beta-glucans, cellulose, and hemicellulose, all found in plant foods. Dietary fibers cannot be digested and absorbed by the small intestine; hence, they end up in the colon, where they are further metabolized by the gut microbiota or excreted. Based on their ability to be soluble in water, dietary fibers are further categorized into soluble and insoluble, exhibiting diverse physiological effects. Carbohydrate intake recommendations To clarify the recommended dietary carbohydrate intake, the Food and Nutrition Board of the Institute of Medicine released guidelines for dietary reference intakes (DRIs), encompassing recommendations for carbohydrate consumption. According to these guidelines, 45-60% of the daily calories should be obtained from carbohydrates, with added sugars not exceeding 10% of the total daily calorie intake. Based on the average glucose requirement for brain function, the minimum carbohydrate intake was set at 130 g/d for both adults and children, with adjusted values for pregnant and lactating women. Recommendations regarding fibers suggest an intake of 25-30 g/d for adults, while the target intake is lower in children. Carbohydrate metabolism and stores After a meal, carbohydrates are broken down to glucose, the primary fuel for energy needs. Once glucose has entered the circulation and uptaken by the body's tissues, it undergoes a series of complex enzymatic and biochemical reactions. This process finally facilitates the adenosine triphosphate (ATP) synthesis, the primary energy unit within the cells. In case of a glucose surplus arising from ingested calories surpassing energy requirements, glucose is stored in the liver and muscles in the form of glycogen or converted into fat in the liver and adipose tissue. On the other hand, fasting periods, for example, during sleep or energy-demanding activities, may necessitate the mobilization of glycogen as the circulating glucose can shortly be depleted. Carbohydrate utilization at rest and during exercise Typically, during rest periods, the organism demands the necessary amounts of carbohydrates to sustain its proper functioning and homeostasis. Therefore, although energy expenditure is relatively low and the main energy substrate contributing to energy expenditure is fat, carbohydrates are still required for the optimal function of the brain, kidneys, reproductive system, and other vital systems. As already mentioned, under normal circumstances, the contribution of carbohydrates to energy production is minimal, as fats constitute the main energy substrate. The way the body utilizes energy substrates (fats and carbohydrates) at rest may be altered in case of metabolic disorders, such as obesity, diabetes, metabolic syndrome, etc., leading to an increased reliance on carbohydrates for energy production. This is due to impaired insulin action (hyperinsulinemia and insulin resistance) and glucose metabolism (hyperglycemia) related to such metabolic disturbances. As a result, fat oxidation is disrupted since a permanent surplus of glucose in the blood is ready to be oxidized for energy production. Thus, the body shifts towards an increased utilization of carbohydrates for energy production. During physical activity, three major energy systems are activated in order to produce ATP, meaning the energy required to drive and support exercise: phosphocreatine (the phosphagen energy system), the aerobic oxidative system, and the anaerobic lactic (glycolytic) energy system. The phosphocreatine energy system is immediately activated in the first 1-10 seconds of high-intensity exercise like sprints, track cycling, weightlifting, etc. This system utilizes the most readily available energy source, phosphocreatine (PCr). It is unable, though, to provide sufficient energy in the case of high-intensity exercise that lasts beyond 10 seconds (approximately 30 seconds-2 minutes). Therefore, the activation of the glycolytic system is necessary. The glycolytic energy system offers the required energy by oxidizing glucose and glycogen. Overall, the combination of the two systems is activated during resistance (high-intensity explosive exercises of decreased duration) and high-intensity interval (HIIT) exercise due to the elevated demand for immediate and sustained ATP production. Conversely, the aerobic oxidative energy system is the long-term one activated in the case of low-to-moderate-intensity continuous endurance exercise, as the previous systems cannot provide the energy fuels needed for extended physical activity. Exercise intensity is one of the primary parameters determining the utilization of carbohydrates as an energy substrate. In low-to-moderate intensity endurance exercise, such as running, rowing, cycling, etc., where the intensity ranges between 50-75% of an individual’s VO2max or 60-80% of their heart rate peak, carbohydrates contribution in energy production is about 30-40%, meaning fat still remains the primary energy fuel. As the intensity of endurance exercise increases, meaning at exercise intensities >70% VO2max or > 80% of the heart rate peak, carbohydrates become the predominant energy fuel, accounting for up to 70% of the total energy expenditure. Pre-workout carbohydrate recommendations With glycogen stores representing only about 5% of the total energy storage, endogenous carbohydrates may not be adequate for prolonged moderate-to-high-intensity exercise. Therefore, consuming a pre-exercise meal rich in carbohydrates ensures both the accessibility of an immediate energy source (glucose) and optimizing glycogen stores. This aids in ensuring sufficient energy supply during physical activity, considering the carbohydrate oxidation rate, which typically ranges between 30-60 g/h. Studies have demonstrated a beneficial effect on exercise performance and prevention of hypoglycemia by consuming a meal rich in complex carbohydrates 2-3 hours before endurance exercise lasting more than 60 minutes. The ingestion of low glycemic index carbohydrates, such as oats, quinoa, legumes, various fruits, and vegetables, is believed to contribute to preserving euglycemia during periods of exercise owing to the gradual release of glucose in the bloodstream and the steady insulin response. Efficient glycogen replenishment As already mentioned, physical activity, especially prolonged endurance exercise of moderate intensity, leads to depletion of glycogen stores, thus generating fatigue and exhaustion. Therefore, nutritional replenishment should be a cornerstone of all athletes’ recovery regimen. The glycogen resynthesis process begins with physical activity termination and lasts 6 to 8 hours. The optimal carbohydrate intake is estimated at 1.2 g/kg/h. High glycemic index carbohydrates, meaning carbohydrates that cause a prompt spike in blood sugar, have been shown to accelerate the process of glycogen restoration, especially when only short-term recovery is available. This is due to the higher stimulation of insulin response triggered by ingesting high glycemic index carbohydrates, compared to low glycemic index carbohydrates. Despite the potential advantageous effects of high glycemic index carbohydrates in short-term recovery, their potency diminishes in prolonged recovery periods. Moreover, consuming a blend of dietary sources containing both glucose and fructose seems to be the most effective approach to glycogen restoration. Lastly, evidence regarding post-workout nutrition suggests simultaneous adequate ingestion of both carbohydrate and protein amounts for muscle recovery and muscle gain purposes. Particularly, co-ingestion of about 1-2.2 g/kg carbohydrates, along with 0.3-5 g/kg proteins in a post-workout meal, is recommended as the optimal approach for post-exercise recovery. Simple carbohydrates and overall health Carbohydrates, in terms of quality and quantity, play a vital role not only in fueling exercise but in sustaining overall health as well. Increased consumption of foods containing simple carbohydrates, meaning high glycemic index carbohydrates, such as refined grains, sodas, desserts, etc., increases the risk for metabolic diseases, including obesity and type II diabetes. The elevated glycemic index results in a rapid rise in blood glucose and, subsequently, an abrupt and uncontrolled secretion of insulin, causing a condition known as hyperinsulinemia. Chronic hyperinsulinemia can lead to insulin resistance, a condition where insulin’s ability to lower blood glucose levels is impaired, resulting in hyperglycemia and possibly type II diabetes. Type II diabetes is a medical condition characterized by insulin resistance due to both persistent hyperinsulinemia and the body's progressive decline in the body's ability to produce insulin. The excessive consumption of simple sugars is among the primary contributing factors to type II diabetes. Therefore, adjusting diet and modifying the quality of the ingested carbohydrates holds great significance in diabetes management. Obesity is another clinical condition that can arise due to the excessive consumption of simple carbohydrates. The increased consumption of simple carbohydrates diminishes satiety signals by interfering with the regulation of dopaminergic and serotonergic systems in the hypothalamus that are responsible for appetite control. This leads to increased caloric intake and a glucose surplus, which is stored as fat, predominantly in the abdominal area (visceral fat). An increase in body fat, especially visceral fat, is associated with numerous health risks, including obesity, cardiovascular diseases, diabetes, etc. Research has also focused on the relationship between CHO consumption and cancer, with several studies demonstrating that the elevated consumption of simple carbohydrates activates pathways that enhance cancer cell proliferation, resulting in tumor growth. However, the research is still ongoing, and no definite recommendations or conclusions can be made. The health benefits of dietary fiber Conversely to the detrimental effects of simple carbohydrates on metabolic, cardiovascular, and overall health, consuming complex carbohydrates, particularly dietary fibers, exerts significant health-promoting effects. Studies have shown the efficiency of soluble dietary fibers, including beta-glucans, pectins, and inulin, in decreasing blood cholesterol, inhibiting atherosclerosis, and regulating blood glucose levels. Ingesting this type of fiber can also increase satiety and alleviate constipation. Moreover, water-soluble fibers are utilized by the gut microbiome, as they are highly fermentable by the gut bacteria. This process contributes to the production of short-chain fatty acids (SCFA), which serve as an energy substrate, regulate cholesterol synthesis, and exhibit anti-inflammatory and apoptotic properties. In other words, SCFA mediate part of the protective effect of soluble dietary fibers on cardiovascular, metabolic diseases, and cancer. Another type of dietary fiber, resistant starch, is also fermented by gut bacteria, exerting the beneficial effects previously mentioned. It also contributes to regulating appetite while also exhibiting beneficial effects on blood glucose regulation and insulin sensitivity. Resistant starch is primarily formed in starchy food by cooling it after it is cooked. It also exists in raw starchy foods such as unripe bananas. Lastly, insoluble fibers, such as cellulose and hemicellulose, effectively enhance fecal mass, decreasing the stool’s transit time in the intestine. Additionally, they induce satiety, thus contributing to weight loss, and also present anti-inflammatory effects. Overall, carbohydrates are vital macronutrients that constitute major energy fuels, ensuring optimal brain and body function. During physical activity, their contribution shifts, with different energy systems being activated depending on the exercise intensity. Sufficient pre- and post-workout carbohydrate intake is crucial for achieving peak performance, improving strength, and supporting recovery. Furthermore, their impact on overall health has various dimensions. While simple carbohydrate consumption constitutes a central pathophysiologic mechanism for developing metabolic disorders such as obesity and diabetes, consuming complex carbohydrates, especially dietary fibers, provides multiple benefits to overall health. REFERENCES Alghannam AF, Gonzalez JT, Betts JA. Restoration of Muscle Glycogen and Functional Capacity: Role of Post-Exercise Carbohydrate and Protein Co-Ingestion. Nutrients. 2018 Feb 23;10(2):253. DOI: https://doi.org/10.3390/nu10020253 Clemente-Suárez VJ, Mielgo-Ayuso J, Martín-Rodríguez A, Ramos-Campo DJ, Redondo-Flórez L, Tornero-Aguilera JF. The Burden of Carbohydrates in Health and Disease. Nutrients. 2022 Sep 15;14(18):3809. DOI: https://doi.org/10.3390/nu14183809 DeMartino P, Cockburn DW. Resistant starch: impact on the gut microbiome and health. Curr Opin Biotechnol. 2020 Feb;61:66-71. DOI: https://doi.org/10.1016/j.copbio.2019.10.008 Jeukendrup A. A step towards personalized sports nutrition: carbohydrate intake during exercise. Sports Med. 2014 May;44 Suppl 1(Suppl 1):S25-33. DOI: https://doi.org/10.1007/s40279-014-0148-z Ludwig DS, Ebbeling CB. The Carbohydrate-Insulin Model of Obesity: Beyond "Calories In, Calories Out". JAMA Intern Med. 2018 Aug 1;178(8):1098-1103. DOI: 10.1001/jamainternmed.2018.2933 Maino Vieytes CA, Taha HM, Burton-Obanla AA, Douglas KG, Arthur AE. Carbohydrate Nutrition and the Risk of Cancer. Curr Nutr Rep. 2019 Sep;8(3):230-239. DOI: 10.1007/s13668-019-0264-3 Margolis LM, Allen JT, Hatch-McChesney A, Pasiakos SM. Coingestion of Carbohydrate and Protein on Muscle Glycogen Synthesis after Exercise: A Meta-analysis. Med Sci Sports Exerc. 2021 Feb 1;53(2):384-393. DOI: 10.1249/MSS.0000000000002476 Mul JD, Stanford KI, Hirshman MF, Goodyear LJ. Exercise and Regulation of Carbohydrate Metabolism. Prog Mol Biol Transl Sci. 2015;135:17-37. DOI: https://doi.org/10.1016/bs.pmbts.2015.07.020 Ormsbee MJ, Bach CW, Baur DA. Pre-exercise nutrition: the role of macronutrients, modified starches and supplements on metabolism and endurance performance. Nutrients. 2014 Apr 29;6(5):1782-808. DOI: https://doi.org/10.3390/nu6051782 P NPV, Joye IJ. Dietary Fibre from Whole Grains and Their Benefits on Metabolic Health. Nutrients. 2020 Oct 5;12(10):3045. DOI: https://doi.org/10.3390/nu12103045 Soliman GA. Dietary Fiber, Atherosclerosis, and Cardiovascular Disease. Nutrients. 2019 May 23;11(5):1155. DOI: https://doi.org/10.3390/nu11051155 Trumbo P, Schlicker S, Yates AA, Poos M; Food and Nutrition Board of the Institute of Medicine, The National Academies. Dietary reference intakes for energy, carbohydrate, fiber, fat, fatty acids, cholesterol, protein and amino acids. J Am Diet Assoc. 2002 Nov;102(11):1621-30. DOI: https://doi.org/10.1016/S0002-8223(02)90346-9 Wu J, Yang K, Fan H, Wei M, Xiong Q. Targeting the gut microbiota and its metabolites for type 2 diabetes mellitus. Front Endocrinol (Lausanne). 2023 May 9;14:1114424. DOI: https://doi.org/10.3389/fendo.2023.1114424
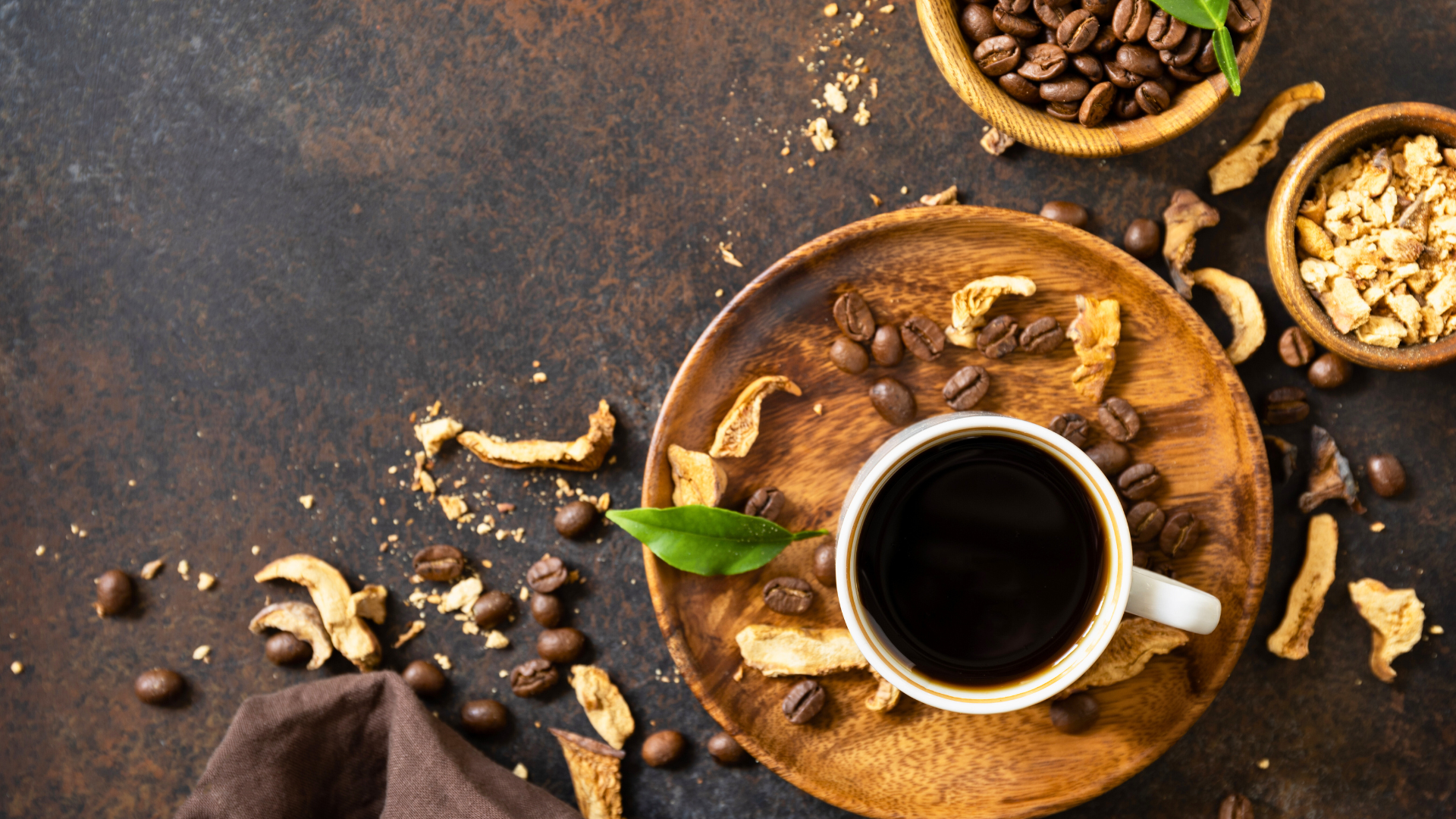
Key points Stimulants belong to three distinct categories, each of which has a different mechanism of action: sympathomimetic amines, psychomotor stimulants, and central nervous system (CNS) stimulants The World Anti-Doping Agency (WADA) has banned the use of some of the most known stimulants, such as amphetamines and ephedrine, by professional athletes Stimulants are used by elite athletes for performance-enhancing purposes, to mitigate physical and mental fatigue as well as to lose weight ahead of competition in sports with weight classes Long-term stimulant use can induce serious health complications related to major body systems, such as the heart and the brain The number of athletes, especially at top levels of competition, as well as the general population reported to be using stimulants, has markedly increased in recent years. The term stimulants covers a broad class of substances directly affecting the central nervous system (CNS). Many individuals use these drugs for various reasons, including performance enhancement, medical benefits, and recreational purposes. They may be legal or illegal. Since one of the primary mechanisms through which stimulants exert their effects is increased blood flow and heart rate, cardiac dysfunction is one of the main concerns associated with their use, along with other adverse effects that will be discussed later. Therefore, in the following article, you will find information about the main stimulant classes and their way of action, the constitutional rules around their use, the negative effects regarding their use, contraindications, and finally, a short overview of the most popular ones. Stimulants classification According to the International Olympic Committee (IOC), stimulants are classified as sympathomimetic amines, psychomotor stimulants, and central nervous system (CNS) stimulants. Sympathomimetic amines mimic or potentiate the effects of the sympathetic nervous system (SNS) through the neurotransmitter norepinephrine. Psychomotor stimulants, such as amphetamines, cocaine, and caffeine, have several effects related to mental function and behaviour, including excitement and euphoria, motor activity increase, and fatigue mitigation. CNS stimulants increase the activity of the CNS's respiratory and vasomotor centres and reflexes. Stimulant usage by athletes Stimulants exert multiple effects pursued by elite and professional athletes. For example, athletes competing in aesthetic sports, such as artistic gymnastics, or in sports with specific weight classes, such as wrestling, may seek stimulant prescriptions for a weight loss advantage. Other athletes competing in team sports, like basketball and football, seek stimulants for increased alertness as well as reduced and delayed fatigue. Other athletes not only use stimulants for performance-enhancing but for recreational purposes as well. Rules around stimulant use The primary method of administration for stimulants is oral intake. Recreational administration of stimulants also occurs by intramuscular and/or intravascular injection, smoking, and intranasal administration. Stimulants can be found in their pure form or over-the-counter sports products, such as pre-workout supplements. In any case, since there is confusion around the rules and recommendations for stimulant use by athletes, the World Anti-Doping Agency (WADA) only permits athletes to take stimulants if deemed necessary by their physicians for therapeutic use. Therapeutic use of stimulants includes attention deficit hyperactivity disorder (ADHD), narcolepsy, asthma, and nasal and sinus congestion, among others. In this case, elite athletes who compete internationally and whose physicians feel they should continue stimulant use must obtain a Therapeutic Use Exemption (TUE) from WADA. The adverse effects of stimulants Given the harmful effects of stimulants, the existence of an organization like WADA is deemed necessary. Therefore, banned stimulants include amphetamines, methamphetamines, ephedrine, pseudoephedrine, cocaine, and other substances with similar chemical structures and biological effects. Regarding permitted stimulants, they can still induce a broad range of short-term and long-term adverse effects and may be physically dangerous when used by athletes who are pushing their bodies to extremes. Specifically, long-term stimulant use can result in decreased appetite and weight loss, headaches, anxiety, insomnia, and shortness of breath. More severe health effects include psychosis, paranoia, stroke, hypertension or hypotension, arrhythmias, myocardial infarction and sudden cardiac death, seizures, and coma. The major factors influencing these outcomes are the user’s body weight, the specific stimulant used, the dose of the agent taken, and tolerance. There are also numerous relative contraindications to the use of stimulants, including individuals with established cardiovascular disease, severe hypertension, untreated hyperthyroidism, glaucoma, and cardiac arrhythmias. Younger athletes under the age of 12 and pregnant women should also avoid using stimulants. The most known stimulants are shortly reviewed below. Caffeine It is the most commonly used stimulant in the world, employed for recreational as well as performance enhancement purposes. As the most commonly used stimulant, caffeine is found in various drinks and foods, such as tea, coffee, and chocolate. It is consumed habitually in many countries worldwide, given its mild to moderate stimulant effects, which promote alertness and increased energy levels. Caffeine is a relatively safe stimulant. Amphetamines They exert multiple effects, including general and cognitive performance enhancement along with euphoric effects. Their general mechanism of action is the stimulation of catecholamines, specifically norepinephrine and dopamine. These catecholamines lead to increased energy levels, euphoria, increased libido, and higher cognition. Athletes use many medications related to the amphetamine class of drugs for physical performance enhancement, including increased strength, acceleration, anaerobic capacity, time to exhaustion, and maximum heart rates. Still, all these drugs fall under bans by WADA. Methamphetamine, a kind of amphetamine, is a widely trafficked and illegal drug mainly used for recreational purposes. Ephedrine and pseudoephedrine They belong to the sympathomimetic amines class of stimulants whose primary mechanism is increased norepinephrine activity at the adrenergic receptors. They are both used as nasal and sinus decongestants caused by the common cold. Athletes may use over-the-counter formulations containing these substances to improve lung function and lower body strength and power before exercise. Cocaine It belongs to the psychomotor stimulants and acts through the blockade of the dopamine transporter protein, resulting in increased dopamine levels. It can temporarily increase energy levels, focus, alertness, and confidence, effects pursued by professional athletes who use it. However, in the long term, cocaine can only harm athletic performance since its use is associated with sleep disruptions, fatigue, anxiety, mood swings, reduced focus, arrhythmias, and hypertension, among others. Cocaine is also used for recreational purposes. Overall, any performance enhancement that an athlete may receive from taking a stimulant raises an important ethical concern. An essential value in sports is fair competition. Athletes should play by the same rules and perform without external influences that may favour them. At high levels of competition, a performance advantage of even one hundred of a second can make a significant difference in first place, opportunities, and financial earnings. Applying this argument to the professional sports field, no use of performance-enhancing substances is fair and, therefore, should be prohibited. References 1. Avois L, Robinson N, Saudan C, Baume N, Mangin P, Saugy M. Central nervous system stimulants and sport practice. Br J Sports Med. 2006;40(Suppl1):i16-i20. DOI: 10.1136/bjsm.2006.027557 2. Berezanskaya J, Cade W, Best TM, Paultre K, Kienstra C. ADHD prescription medications and their effect on athletic performance: A systematic review and meta-analysis. Sports Med-Open. 2022;8(1):5. DOI: 10.1186/s40798-021-00374-y 3. Farzam K, Faizy RM, Saadabadi A. Stimulants. In: StatPearls. StatPearls Publishing, Treasure Island (FL);2022. DOI: 30969718 4. Garner AA, Hansen AA, Baxley C, Ross MJ. The use of stimulant medication to treat Attention-Deficit/Hyperactivity Disorder in elite athletes: A performance and health perspective. Sports Med. 2018;48(3):507-512. DOI: 10.1007/s40279-017-0829-5 5. Reardon CL, Factor RM. Considerations in the use of stimulants in sport. Sports Med. 2016;46(5):611-617. DOI: 10.1007/s40279-015-0456-y
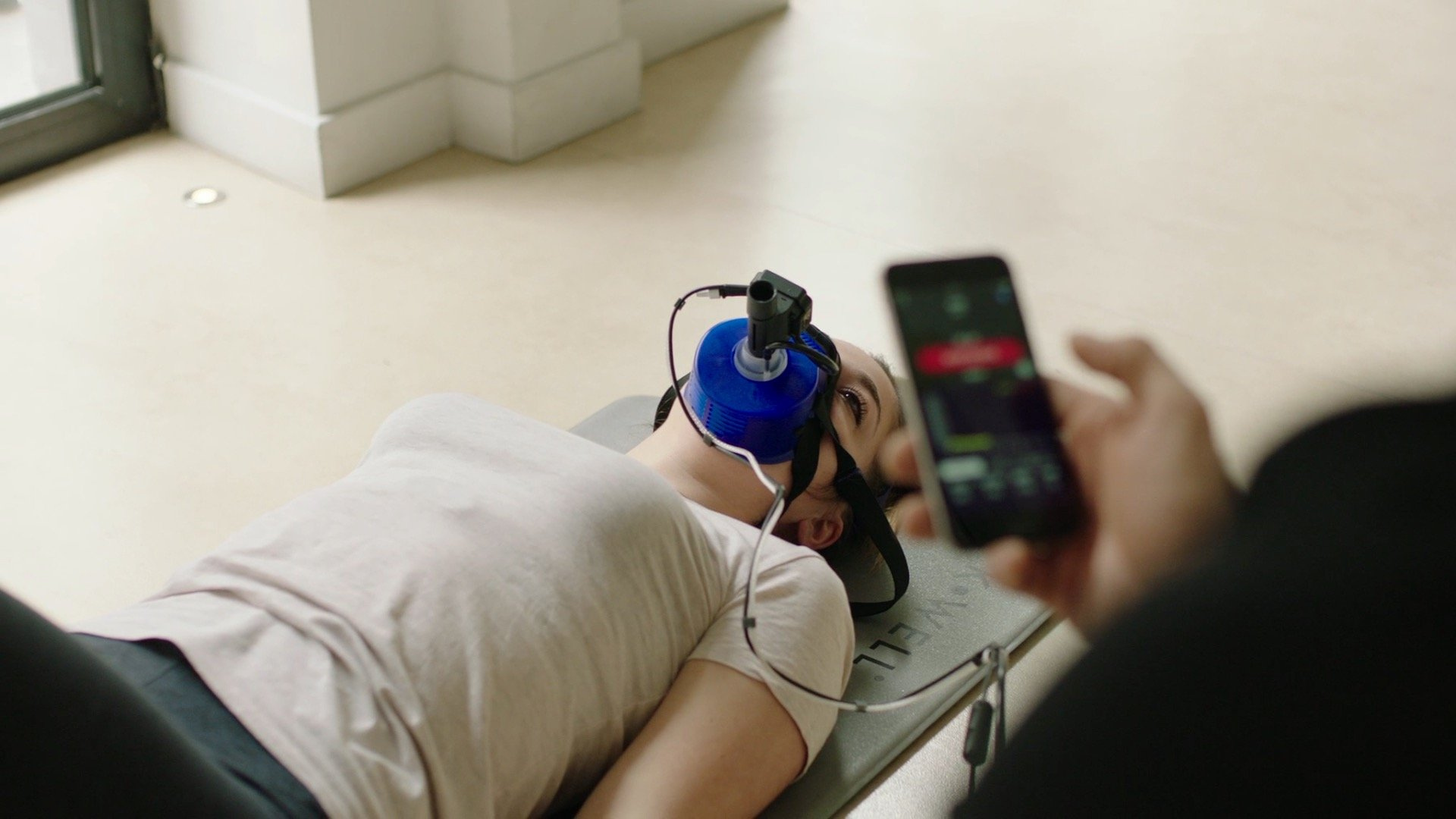
Diabetes is as common as it is misunderstood. Perhaps the most common misconception is that it’s a consequence of carbohydrate overconsumption. Perhaps the most straightforward proof that diabetes is not a result of carbohydrate consumption is the fact that humans consumed significantly more carbohydrates in previous centuries without triggering anything close to the diabetes epidemic our world faces today. Clearly, the fact that diabetes was practically nonexistent before the 1950s but has exponentially propagated in just a few decades indicates that it constitutes a much more complex metabolic dysfunction that is inextricably linked to our modern way of life. This article explains the pathophysiological origins of diabetes and its link to chronic inflammation and fat accumulation. A comprehensive 7-step overview explores how inflammation causes insulin resistance, leading to fat accumulation, low energy levels, and, ultimately, diabetes. Last, we discuss how the progression of this condition can be monitored reliably through breath analysis. Step 1 - Onset of inflammation Exogenous deleterious factors stimulate the production of inflammatory substances in our body’s response to mitigate the negative impact such factors may induce. Inflammatory cytokines, such as tumour necrosis factor-alpha (TNF-α) and interleukin-6 (IL-6), can activate various signalling pathways that interfere with insulin signalling. This disruption often involves inhibiting insulin's ability to promote glucose uptake and utilization in cells, leading to insulin resistance. Some of the most prominent inflammatory factors include: Excessive calorie consumption Junk food Lack of micronutrient intake Lack of physical exercise Lack of sleep In our recent article about inflammation, we dive deeper into the exogenous stimuli that trigger our body’s inflammatory responses and the principal prevention mechanisms we can apply daily. Step 2 - Inflammation neutralizes our cells’ insulin receptors (Insulin Resistance) Inflammatory markers exert their detrimental effects on insulin receptors of cells through various molecular mechanisms. These markers initiate inflammatory pathways that interfere with insulin signalling cascades upon activation. This interference includes the increased phosphorylation of serine residues on insulin receptor substrate-1 (IRS-1), diminishing its ability to relay signals downstream in the insulin pathway. Additionally, inflammatory cytokines can directly hinder insulin receptor activity, possibly through receptor internalization or impaired autophosphorylation. Activation of stress-sensitive kinases, such as JNK and IKK, further exacerbates insulin resistance by phosphorylating IRS-1, impeding its interaction with downstream signalling molecules. Furthermore, inflammatory signals disrupt insulin-stimulated glucose transporter type 4 (GLUT4) translocation to the cell membrane, consequently reducing glucose uptake. These molecular disruptions culminate in insulin resistance, a pivotal factor in the pathogenesis of conditions like type 2 diabetes, where chronic low-grade inflammation plays a significant contributory role. Step 3 - Insulin resistance causes fuel utilization dysfunction When we consume food, our pancreas responds by secreting insulin, the hormone that enables glucose to enter cells and be oxidized. When cells, including muscle cells, become insulin resistant, there's an impaired ability to use glucose for energy efficiently. Cellular desensitization to insulin means that they no longer respond to insulin and are thus unable to absorb and utilize glucose. To compensate for this reduced glucose utilization, there is an increased reliance on alternative energy sources, such as fatty acids. Step 4 - Fuel utilization dysfunction leads to fat accumulation and lower energy levels In insulin-resistant states, cells, especially muscle cells, enhance their uptake of fatty acids and prioritize lipid storage over glucose utilization. This shift towards increased fatty acid uptake and storage contributes to the accumulation of intramyocellular lipids (i.e., the buildup of fat within our muscles and organs). Essentially, when cells become insulin resistant, they favour fat accumulation because their ability to respond to insulin's signals for glucose uptake properly is compromised. Since glucose is the primary energy source for cells, their inability to absorb it deprives them of the valuable fuel they need, leading them to develop feelings of fatigue and low energy levels. Step 5 - Accumulated fat causes more inflammation and insulin resistance Both visceral fat, found around internal organs in the abdominal cavity, and intramyocellular fat, which accumulates within muscle cells, are sources of inflammatory markers. Visceral fat is highly metabolically active and secretes pro-inflammatory cytokines and adipokines such as TNF-alpha, IL-6, and leptin. These substances contribute to chronic low-grade inflammation, a key factor in developing conditions like insulin resistance and cardiovascular diseases. Similarly, intramyocellular fat accumulation disrupts cellular processes and is associated with increased production of inflammatory markers. TNF-alpha, IL-6, and other cytokines released by intramyocellular fat can impair insulin signalling within muscle cells, further contributing to metabolic dysfunction and insulin resistance. Overall, both visceral and intramyocellular fat play roles in systemic inflammation through the secretion of inflammatory markers, which have significant implications for metabolic health and the development of chronic diseases. Step 6 - Insulin resistance leads to elevated blood sugar (Pre-diabetes) In insulin resistance, cells become less responsive to insulin signals, particularly muscle, fat, and liver cells. Typically, insulin facilitates glucose uptake into these cells by promoting the translocation of glucose transporter proteins, such as GLUT4, to the cell membrane. However, this process is impaired in insulin resistance, resulting in reduced glucose uptake by cells, particularly in muscle and fat tissues. Consequently, less glucose is taken from the bloodstream and sent into cells for energy use. Step 7 - Pancreas goes on over-drive and ultimately fails (Diabetes) Initially, the body attempts to compensate for insulin resistance by producing more insulin (hyperinsulinemia) to overcome the decreased responsiveness of cells. This compensatory mechanism helps maintain relatively normal blood sugar levels in the early stages of insulin resistance. However, over time, the pancreas may fail to sustain this increased insulin secretion, leading to a decline in insulin production and exacerbating hyperglycemia. Breath analysis, an easy and reliable monitoring tool for metabolic function. As described in the 7-step process above, the fundamental consequence that unilaterally describes metabolic dysfunction is the cells’ inability to metabolize glucose and instead favours nutrient storage overutilization for energy production. Simply put, when consuming food, metabolic impaired individuals store it instead of using it to power the body. Measuring the Respiratory Exchange Ratio (RER), the balance between carbon dioxide production over oxygen consumption during the post-prandial state (i.e., after a meal), is perhaps the easiest and most reliable method for understanding whether our cells can utilize the food we consume. In metabolically healthy individuals, the respiratory exchange ratio will rise precipitously after food consumption, indicating that cells absorb and use the nutrients ingested. Conversely, in metabolically compromised individuals, RER will exhibit a blunt increase, indicating that nutrients cannot enter cells, get oxidized, and produce carbon dioxide that would otherwise cause RER to rise. Breath analysis not only provides a direct measure of the fundamental mechanism defining metabolic dysfunction but also constitutes a non-invasive and easy assessment. This is in contrast to traditionally used methods such as the euglycemic insulin clamp, which requires trained medical professionals to perform blood analysis and be present at a medical facility.
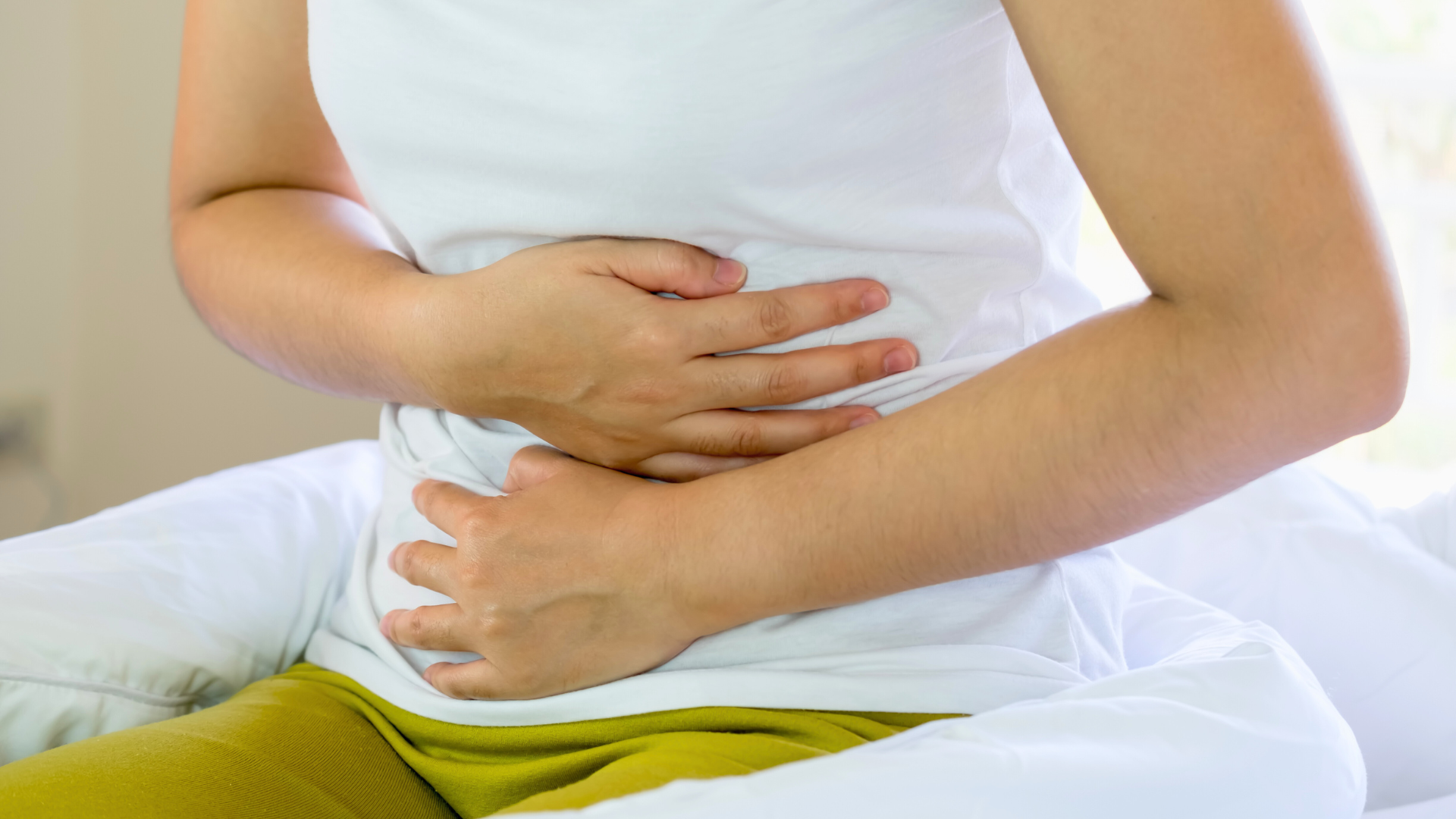
Key points Inflammation is a physiological, tightly regulated, protective process in response to harmful stimuli, such as pathogens, trauma, chemicals, etc. Chronic inflammation is associated with the development of severe chronic health issues, such as cardiovascular disease, type II diabetes, cancer, neurodegenerative and autoimmune diseases The most important causative factors of chronic inflammation are obesity and specifically visceral fat, stress, sleep disturbances, environmental chemicals, and unhealthy dietary constituents and patterns Regular exercise, adherence to healthy dietary patterns, and mind-body interventions have the potential to decrease or even reverse chronic inflammation Over the last decade, there has been much discussion about inflammation and whether there is such a thing as chronic inflammation, as well as anti-inflammatory agents in terms of food and botanical constituents that could effectively battle it. It seems that it all adds up since chronic inflammation does exist and is at its peak rate, probably due to the contemporary lifestyle. In this post, topics such as what inflammation is as a biological process and what may cause it, its relation to lifestyle factors such as diet, exercise, and environmental chemicals, as well as its implication with chronic severe diseases such as obesity, type II diabetes, and cardiovascular disease will be discussed. What really inflammation is and how is it caused Inflammation is a physiological, tightly regulated, protective process in response to harmful stimuli. Insults that can trigger inflammation include: Infection from pathogenic microorganisms like bacteria, viruses, or fungi Tissue damage from trauma Necrotic cells of human tissue remaining after fighting a harmful agent External injuries like scrapes Effects of irritants and toxic compounds like chemicals Irradiation The goal of inflammation is to destroy the harmful stimuli that initiated it and start the repair process, restoring the involved tissues to their pre-inflamed state and thus re-establishing homeostasis. Therefore, acute inflammation, which resolves in a few days after eradicating the inflammatory stimulus, is a beneficial, biologically appropriate process required for regaining tissue homeostasis after damage within the human body has occurred. Symptoms associated with signs of acute inflammation include redness, heat, swelling, pain, and temporary loss of function at the site of inflammation. The acute inflammatory response involves the recruitment of immune system cells, known as leucocytes, such as neutrophils, macrophages, monocytes, etc. These cells release inflammatory mediators, including reactive oxygen species (ROS) and inflammatory cytokines, such as interleukin-1 (IL-1), interleukin-6 (IL-6), and tumor necrosis factor-a (TNF-a) to kill the harmful agent. Since the goal of the acute inflammatory response is threat removal, without specificity or selectivity, collateral tissue damage is the inevitable consequence of massive ROS and cytokine production from leucocytes. Nevertheless, the resolution and self-termination of inflammation and the return to baseline status in days to weeks following the eradication of the inflammatory stimulus ensures survival and is not a pathological response. If this elegant coordination of immune system adaptations fails to resolve or resolves inadequately since the inflammatory stimuli persist or propagate, inflammation can become chronic, self-directed, and thus dangerous. Specifically, the chronicity of inflammation is associated with excessive human tissue damage and various severe disease states, including inflammatory bowel disease, cancer, type II diabetes, heart disease, and autoimmune disorders, such as rheumatoid arthritis, multiple sclerosis, and systemic lupus erythematosus. Although this relationship is very complicated and has not yet been elucidated, possible mechanisms connecting chronic inflammation with multiple health conditions include its association with elevated blood glucose levels and insulin resistance, with sodium, fluid retention, and hypertension, as well as with persistently elevated levels of cytokines such as IL-6 and C-reactive protein (CRP). As mentioned above, chronic low-grade inflammation is associated with the development of severe chronic health issues, some of them being among the top causes of death worldwide, such as cardiovascular disease, type II diabetes, obesity, cancer, and neurodegenerative diseases. It could be actually argued that inflammation is not only associated with the disease condition itself but could also be involved in its pathogenesis and progression. The common feature in these disease states is a silent low-grade inflammatory process, reflected as an increase in systemic plasma concentrations of inflammatory markers, such as cytokines (IL-1, IL-6, TNF-a, CRP, etc.). For instance, higher concentrations of inflammatory markers such as IL-6, TNF-a, and CRP have been shown to be associated with higher cardiovascular risk. So, how can this chronic silent inflammatory process be triggered? Lifestyle factors, including smoking, alcohol consumption, poor diet, low levels of physical activity, exposure to environmental chemicals, and increased stress, contribute to the development of low-grade inflammation. Hence, strategies to improve overall lifestyle, including adhering to a healthy diet, regular exercise, adequate sleep, and social support, may be an effective approach to prevent chronic inflammation by modifying risk factors for chronic diseases associated with it. The most important causative factors of chronic inflammation are discussed below. Obesity and its central role in chronic inflammation The vast majority of human fat tissue is of the white type; this type of fat is mainly located beneath the skin (subcutaneous adipose tissue) and around internal organs (visceral adipose tissue). White adipose tissue is not only an energy storage place but is also metabolically active, regulating several metabolic pathways, including immunity and inflammation. Specifically, adipocytes secrete numerous hormones and cytokines, collectively called adipokines. Some of them are pro-inflammatory, such as the hormones leptin and resistin, and the cytokines IL-6, CRP, and TNF-a, and some of them are anti-inflammatory, such as the hormone adiponectin. While research has shown an association between both central obesity (visceral fat) and total obesity and inflammation, increased visceral fat is the primary source of chronic systemic low-grade inflammation. Although the association is not clear yet, it seems that the link between central obesity and inflammation is the oversecretion of pro-inflammatory adipokines and free fatty acids by the visceral fat of obese individuals. More specifically, obesity is linked to the enlargement of adipocytes (hyperplasia), leading to hypoxic conditions within these cells. As a result, a local inflammatory response is triggered, with the recruitment of immune cells, such as macrophages, and the subsequent accumulation of the pro-inflammatory cytokines IL-6 and TNF-a. IL-6, in turn, stimulates CRP production in the liver and the employment of more immune cells. Simultaneously, since fat tissue has a limited capacity to store energy, once this is exceeded, like in hyperplastic fat cells, lipolysis occurs within the cells, causing a release of free fatty acids into the circulation. Free fatty acids reinforce the release of pro-inflammatory cytokines and also directly mediate the inflammatory process. The association between visceral fat and inflammation is actually proportional, meaning the higher the body weight and the body fat, the higher the levels of the pro-inflammatory adipokines and, therefore, the higher the level of inflammation. It’s thus becoming evident that obesity predisposes to a pro-inflammatory state. Inflammation results in the massive production of ROS, also leading to oxidative stress. Both oxidative stress and inflammation statuses are strongly associated with chronic severe health complications, including cardiovascular disease, insulin resistance, hypertension, type II diabetes, obstructive sleep apnea, rheumatoid arthritis, dementia, and cancer. Therefore, obesity, especially large visceral fat volumes (central obesity), through its chronic inflammatory components, is involved in the pathogenesis and progression of cardiometabolic, neurodegenerative, and autoimmune diseases. Inflammation caused by dysregulated sleep patterns Lifestyle behaviours like sleep have been linked to heightened inflammatory responses. Moreover, sleep issues have been associated with an increased risk of multiple inflammatory disorders, including cardiovascular disease and neurodegenerative diseases. So, could chronic inflammation be the link connecting sleep issues with adverse public health outcomes? Indeed, sleep disturbances in terms of sleep deprivation, insomnia, sleep restriction (sleeping less than 5 hours per night), and sleep fragmentation (nocturnal waking for ≥ 90 minutes) lead to increased inflammation due to changes in the immune system that trigger inflammatory responses. A possible mechanism is that sleep disturbances induce a shift in the temporal profile of inflammatory responses, with increased production of the pro-inflammatory cytokines IL-6 and TNF-a during the day rather than during the night, leading to excessive levels of inflammation. Due to the increased production of IL-6, there is also a subsequent overproduction of CRP, further propagating inflammation. If the sleep disturbance is persistent, it leads to sustained activation of the inflammatory response and, thus, chronic inflammation. Inflammation caused by chronic stress Chronic stress in major life domains (relationships, work, finances) stimulates chronic inflammation in both men and women, which is reflected in the elevated CRP levels. Additionally, emerging research suggests that social support and network may have a role in mitigating the psychological impact of major life stressors, thus attenuating their potential to cause chronic inflammation. Moreover, mind-body interventions such as tai chi and meditation are emerging as promising strategies to reduce stress and thus decrease or even reverse inflammation, with effects on the severity or even the prevention of pathologies related to chronic inflammation, such as neurodegenerative and autoimmune diseases. Inflammation caused by environmental chemicals Chemical exposure in the environment, including long-term exposure to polycyclic aromatic hydrocarbons (PAHs), perfuoroalkyl substances (PFAs), and metal exposure, is responsible for causing chronic inflammatory responses. PFAs have significant bioaccumulation potential and are widely used in food packaging, household cleaning products, cosmetics, etc. PAHs are a group of chemicals formed during the incomplete combustion of coal, oil, gas, and garbage, including vehicle exhaust, coal tar, wildfires, agricultural burning, etc. Regarding metal exposure, arsenic is a toxic metal widely distributed in the environment and is present in soil, food, and water, leading to unavoidable human exposure. Cadmium is mainly released from nickel-cadmium batteries, plastic stabilizers, fossil fuel combustion, and garbage incineration. Mercury pollution primarily comes from burning coal, non-ferrous metals, and cement production. All these environmental pollutants can enter the human body through the respiratory tract, digestive tract, and skin and interact with the immune system, inducing a chronic inflammatory response and, thus, the possibility of chronic inflammatory diseases, such as cancer and autoimmune disorders. The implication of exercise with chronic inflammation Physical inactivity is one of the most important lifestyle factors associated with persistent systemic low-grade inflammation and, thus, an increased likelihood of inflammatory diseases. On the other hand, regular exercise possesses anti-inflammatory effects, thereby reducing disease risk. The anti-inflammatory effects of regular exercise are attributed to several mechanisms, the most critical of which are increased fat oxidation and reduced visceral body fat stores. Specifically, exercise results in an increased skeletal muscle capacity to burn fat, resulting in increased fat oxidation in mitochondria and decreased overall lipid storage inside cells. Consequently, exercise helps limit visceral fat accumulation and adipose tissue expansion. Since fat tissue and visceral fat, in particular, are metabolically active and release pro-inflammatory mediators, as stated before, exercise limits inflammation activation by downregulating these pro-inflammatory mediators, including cytokines. Specifically, it has been shown that regular exercise reduces the levels of IL-1 and IL-6. Moreover, active skeletal muscles secrete molecules known as myokines, which help counterbalance the pro-inflammatory effects of cytokines. Since the inflammatory effects of physical inactivity run mostly through its impact on visceral fat and obesity, it could be supported that a link between physical inactivity, visceral fat accumulation (central obesity), and inflammation likely exists. However, the association between chronic systemic inflammation and physical inactivity is independent of obesity status. Collectively, regular physical activity and its associated fat loss may offer prevention and treatment for various chronic diseases associated with low-grade inflammation. It is inexpensive and without the side effects of many pharmacological therapies and could be viewed as a natural remedy for recovering part of the inflammatory burden caused by modern lifestyles. The implication of diet with chronic inflammation Primarily, nutrition serves as the source of essential nutrients, providing energy and substrates for numerous metabolic functions. In cases of obesity and, thus, chronic inflammation, a dietary pattern encompassing caloric restriction has been proven effective in reducing inflammation and metabolic dysfunction related to obesity status. Besides the caloric restriction that can reduce chronic inflammation by decreasing visceral fat, several studies demonstrate an inverse association between inflammatory markers and adherence to healthy dietary patterns. Specifically, nutritional factors such as dietary fiber, antioxidants, and omega-3 fatty acids have been associated with decreased concentrations of inflammatory markers. In contrast, dietary factors, such as trans and saturated fat, sugar, and sodium, have been associated with increased levels of inflammation. Dietary fiber Fiber-rich diets are often associated with a high intake of antioxidants and complex carbohydrates, both of which may reduce inflammation. Another anti-inflammatory mechanism of fiber is its conversion into immune-regulating substances, such as short-chain fatty acids, by the gut microbiota in the colon. These substances activate signaling pathways, eventually decreasing the inflammatory response by reducing the pro-inflammatory cytokines IL-6, TNF-a, and CRP production. Polyphenols Polyphenols are a heterogeneous group of bioactive substances found in plant-based foods. They are known to have potent antioxidant and anti-inflammatory effects, thanks to their ability to reduce ROS and the pro-inflammatory cytokines IL-6 and TNF-a, respectively. Omega-3 fatty acids Omega-3 fatty acids such as eicosapentaenoic acid (EPA) and docosahexaenoic acid (DHA) are found in fish and fish oils and are considered anti-inflammatory. They have been shown to improve markers of cardiovascular disease, rheumatoid arthritis, and cancer cachexia, all disease states associated with chronic inflammation. Trans fatty acids Trans fatty acids have predominantly pro-inflammatory properties by activating inflammatory pathways and increasing oxidative stress through increased ROS production. Their primary source is partially hydrogenated oils, usually the result of industrial food processing. They are also partly derived from ruminant animal products. Saturated fat Similarly to trans fatty acids, saturated fat also seems to exert pro-inflammatory effects due to increased production of ROS and activation of pro-inflammatory pathways. Sugar Food products with high levels of free-added sugar seem to have enhanced pro-inflammatory effects and may be linked to the development of chronic diseases associated with inflammatory processes, such as atherosclerosis, cancer, and Alzheimer’s disease. A possible explanation is a chronic and exaggerated increase in blood glucose caused by such foods, which can lead to the excessive formation of advanced glycation end products (AGEs). AGEs may cause oxidative stress and trigger inflammatory responses. Dietary patterns High adherence to the Mediterranean diet or the DASH (Dietary Approaches to Stop Hypertension) has been associated with decreased CRP, IL-6, and TNF-a levels, as well as oxidative stress biomarkers. The high content of anti-inflammatory nutrients such as omega-3 fatty acids, dietary fiber, complex carbohydrates, and polyphenols may explain the consistent anti-inflammatory effects of such diets, which are rich in fruits, vegetables, legumes, and whole grains. Also, adherence to a Paleolithic diet, rich in plant-based and non-processed animal products but low in processed foods, added sugars, salt, and dairy, has also been linked to a decrease in inflammation markers, especially CRP and oxidative biomarkers. In contrast, the ‘’Western’’ dietary pattern rich in processed meats, refined grains, and sugary beverages is linked to increased inflammatory markers. To sum up, the battlefronts of chronic inflammation are multiple, and if silently working chronically without us making lifestyle changes to decrease them or even completely eradicate them, they can lead to severe health issues that can compromise quality of life and reduce lifespan. However, the ability of inexpensive and undemanding remedies, such as diet, exercise, meditation, etc., to effectively combat chronic inflammation is in front of our eyes and the palm of our hands, so it should not be neglected. References 1. Alexopoulos N, Katritsis D, Raggi P. Visceral adipose tissue as a source of inflammation and promoter of atherosclerosis. Atherosclerosis. 2014;233(1):104-112. DOI: 10.1016/j.atherosclerosis.2013.12.023 2. Bruckner F, Gruber JR, Ruf A, Thanarajah SE, Reif A, Matura S. Exploring the link between lifestyle, inflammation, and insulin resistance through an improved Healthy Living Index. Nutrients. 2024;16(3):388. DOI: 10.3390/nu16030388 3. Burini RC, Anderson E, Durstine JL, Carson JA. Inflammation, physical activity, and chronic disease: An evolutionary perspective. Sports Med Health Sci. 2020;2(1):1-6. DOI: 10.1016/j.smhs.2020.03.004 4. Ellulu MS, Patimah I, Khaza’ai H, Rahmat A, Abed Y. Obesity and inflammation: the linking mechanism and the complications. Arch Med Sci. 2017;13(4):851-863. DOI: 10.5114/aoms.2016.58928 5. Hess JM, Stephensen CB, Kratz M, Bolling BW. Exploring the links between diet and inflammation: Dairy foods as case studies. Adv Nutr. 2021;12(Suppl1):1S-13S. DOI: 10.1093/advances/nmab108 6. Irwin MR, Sleep and inflammation: partners in sickness and in health. Nat Rev Immunol. 2019;19(11):702-715. DOI: 10.1038/s41577-019-0190-z 7. Khanna D, Khanna S, Khanna P, Kahar P, Patel BM. Obesity: A chronic low-grade inflammation and its markers. Cureus. 2022;14(2):e22711. DOI: 10.7759/cureus.22711 8. Liu Y, Zhang Z, Han D, Zhao Y, Yan X, Cui S. Association between environmental chemicals co-exposure and peripheral blood immune-inflammatory indicators. Front Public Health. 2022;10:980987. DOI: 10.3389/fpubh.2022.980987 9. Oronsky B, Caroen S, Reid T. What exactly is inflammation (and what is it not?). Int J Mol Sci. 2022;23(23):14905. DOI: 10.3390/ijms232314905 10. Ricordi S, Garcia-Contreras M, Farnetti S. Diet and inflammation: Possible effects on immunity, chronic diseases, and life span. J Am Coll Nutr. 2015;34Suppl1:10-13. DOI: 10.1080/07315724.2015.1080101 11. Sotos-Prieto M, Bhupathiraju SN, Falcon LM, Gao X, Tucker KL, Mattei J. Association between a Healthy Lifestyle Score and inflammatory markers among Puerto Rican adults. Nutr Metab Cardiovasc Dis. 2016;26(3):178-184. DOI: 10.1016/j.numecd.2015.12.004 12. Stumpf F, Keller B, Gressies C, Schuetz P. Inflammation and nutrition: Friend or foe? Nutrients. 2023;15(5):1159. DOI: 10.3390/nu15051159
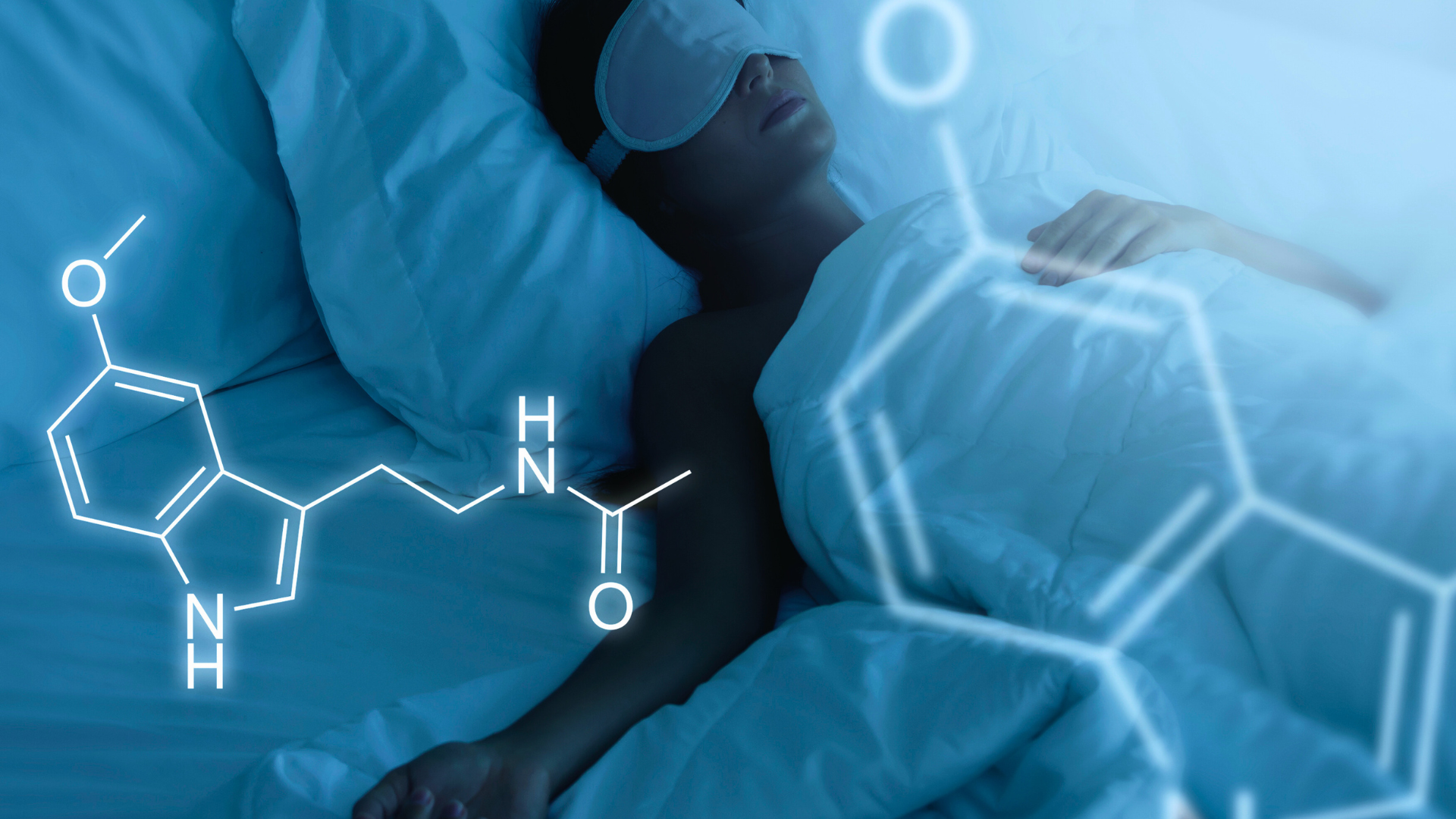
Key Points Sleep deprivation disrupts glucose metabolism and the typical secretion patterns of the appetite hormones, contributing to metabolic dysfunctions. Sleep hygiene is a valuable tool for cardiovascular disease prevention. Poor sleep quality shifts the autonomic nervous system balance towards sympathetic dominance, increasing the risk for cardiovascular disorders. The circadian rhythms of cortisol, adrenaline, and melatonin influence cardiovascular parameters, with disruptions due to sleep deprivation compromising cardiovascular health. Our previous article about sleep discussed basic sleep concepts, such as sleep quality and duration, and their impact on recovery and exercise performance. In closing the chapter about sleep and its broad influences on overall health and well-being, the impact of sleep on metabolic and cardiovascular health will be analyzed in the second part. Metabolic health aspects, such as glucose regulation, appetite hormones, and energy balance, as well as cardiovascular health aspects, such as autonomic nervous system regulation and hormones, will be considered. Sleep and metabolic health Glucose regulation In healthy individuals, glucose regulation appears to be under circadian control. Particularly, insulin sensitivity and glucose tolerance, which are major regulating factors of glucose metabolism, tend to be elevated during mornings and decrease as the day progresses. Circadian misalignment induced by sleep deprivation, poor sleep quality, or irregular sleeping patterns can disrupt these abilities, resulting in elevated blood glucose levels and extended time to restore normal levels. Thus, the chronically elevated blood sugar levels associated with systematic sleep deprivation can result in insulin resistance, leading to a high risk of developing a plethora of metabolic disorders, such as type II diabetes, obesity, and metabolic syndrome. Indicatively, robust data from experimental sleep restriction indicated that individuals with short sleep durations (≤6–7 h/night) are at 30% greater risk for developing type II diabetes. Appetite hormones and energy balance Another aspect of metabolism associated with circadian misalignment due to sleep disturbances is the 24-hour variation of the appetite hormones leptin and ghrelin. Leptin and ghrelin are primary coordinators of energy intake and energy expenditure throughout the day. Leptin is the satiety hormone that maintains energy balance by reducing caloric intake through decreased appetite, deterring fat production, and increasing energy expenditure. On the other hand, ghrelin is considered an orexigenic hormone, secreted mainly by the stomach. Ghrelin also plays a fundamental role in energy balance regulation with an opposite to leptin function. Their functions are synchronized with leptin levels, which typically rise during the early stages of sleep and reach their lowest early in the morning, while ghrelin follows an inverse pattern. Ghrelin declines during bedtime and reaches its peak early in the morning, remaining high before meals and typically returning to baseline postprandially. Although further research is required to elucidate the exact mechanisms, existing studies suggest that poor sleep quality and sleep deprivation interfere with appetite hormones’ secretion by altering the timing of their secretion, thus leading to dysregulation. Particularly, sleep restriction and irregular sleeping patterns are associated with diminished secretion of leptin and increased secretion of ghrelin, promoting elevated caloric intake and subsequent weight gain. From the evidence obtained so far, there might be a link between short sleep duration, adiposity, and the development of metabolic disorders. The primary mechanism proposed for the dysregulation of appetite hormones due to sleep abnormalities is that sleep deprivation acts as a chronic stressor, affecting the hypothalamic-pituitary-adrenal axis function. The hypothalamic–pituitary–adrenal axis is responsible for cortisol production and secretion, an aforementioned stress hormone with catabolic properties. Sleep deprivation-induced stress can lead to elevated cortisol secretion by the brain, interfering with the normal signaling of the appetite hormones. As a consequence, leptin’s sensitivity is diminished, and even when the body has adequate energy reserves, the individual does not experience satiety. Additionally, cortisol can stimulate ghrelin secretion, further boosting feelings of hunger and potentially leading to weight gain. Overall, sleep deprivation can have adverse effects on various aspects of metabolic health by disrupting glucose regulation, inducing stress, and potentially altering appetite control, resulting in a heightened risk of metabolic disorders like diabetes, type II diabetes, obesity, and metabolic syndrome. Sleep and cardiovascular health Autonomic Nervous System’s adaptations during healthy sleep Adequate and quality sleep is considered fundamental in preserving cardiovascular health, as underlined in the recent guidelines released by the American College of Cardiology/American Heart Association. These guidelines state that counseling on sleep and its hygiene is a valuable approach to cardiovascular disease (CVD) prevention. This importance can be attributed to the vital rest period sleep offers the cardiovascular system. Particularly, during sleep, cardiovascular regulation is modified, and many biological functions slow down, allowing the heart to rest from the stress of waking hours and facilitating efficient restoration of the cardiovascular tissues. In this context, the autonomic nervous system (ANS), which controls physiological body processes that occur despite our control, plays a central role. Specifically, a suppression of the sympathetic nervous system and increased parasympathetic system activity occurs. In other words, our body transitions from an alert state, often called the «fight or flight» response, to a state of relaxation and rejuvenation. This shift is predominantly evident during stages N1, N2, and N3 of NREM sleep. During NREM sleep, heart rate and arterial blood pressure tend to decrease. This is because a shift towards a more parasympathetic tone occurs, promoting vasodilation (widening of blood vessels), enhancing blood flow to peripheral tissues, and thus fostering relaxation and tissue repair. On the contrary, during REM sleep, which involves the transition from sleep to wakefulness, our nervous system is predominantly under sympathetic control, leading to elevated heart rate and arterial blood pressure values. The interplay between the sympathetic and parasympathetic activities can be efficiently reflected by HRV, a marker that serves as an indicator of the fluctuations in the time intervals between consecutive heartbeats. HRV normal values differ between populations and depend on factors like age, gender, and fitness level. During sleep, higher HRV values, meaning broader time variation between heartbeats, are associated with balanced autonomic nervous system activity and resilience to stress. Conversely, lower HRV indicates autonomic dysregulation, diminished adaptivity between states of alertness and relaxation, and potential susceptibility to cardiovascular issues. Autonomic Nervous System’s adaptations during sleep deprivation It’s becoming clear that the interplay between the two major branches of the autonomic nervous system (the sympathetic and parasympathetic nervous systems) is of great importance in achieving restful sleep. Disruptions of sleep duration and quality appear to disrupt this interplay, resulting in considerable predominance of the sympathetic nervous system’s activity. This suggests that sleep deprivation impairs the nervous system’s ability to adapt to shifting toward relaxation mode, subjecting individuals to a prolonged state of alertness and stress. As a result, a cascade of events occurs, including lower HRV values, increased proinflammatory cytokine secretion, and vasoconstriction, increasing the risk of hypertension, coronary artery disease, endothelial dysfunction, and other cardiovascular disorders. Circadian Hormonal Dynamics and Cardiovascular Health The circadian rhythm has implications for the cardiovascular system, as well. Hormones like cortisol, melatonin, and adrenaline, which follow circadian patterns, play a critical role in cardiovascular regulation. As mentioned before, cortisol is a stress hormone, and its levels typically rise in the morning and reach their lowest during the night, enabling the anabolic hormones to facilitate recovery. However, chronic sleep deprivation can alter this hormonal synchronization, leading to dysregulated cortisol levels, potentially contributing to increased blood pressure and consequent risk of cardiovascular diseases. Likewise, adrenaline, another hormone typically released as a response to stress stimuli, peaks throughout the day when the individual is active and the sympathetic nervous system governs. Sleep deprivation and irregular sleeping patterns can result in circadian misalignment associated with dysregulation of adrenaline secretion. This dysregulation leads to sympathetic nervous system dominance and, thus, elevated heart rate and blood pressure, meaning an increased risk for cardiovascular disorders. Finally, melatonin, a hormone released by the brain, typically rises during the night as sleeping time approaches. Melatonin is known to exert cardioprotective effects through its antioxidant, anti-inflammatory, antiatherogenic, and lipid-lowering properties. Moreover, melatonin regulates blood pressure and vascular tone by contributing to blood pressure reduction during sleep. Hence, disruptions in melatonin secretion, often originating from chronic insufficient or poor sleep, pose a non-negligent risk for cardiovascular diseases. To sum up, adequate sleep quality is a good indicator of metabolic and cardiovascular health. Therefore, prioritizing sufficient and quality sleep for the optimal functioning of the cardiovascular and metabolic health system and thus for longevity is crucial and should not be ignored since sleep is, along with nutrition and exercise, amongst the main pillars of a healthy, long life. REFERENCES Baranwal N, Yu PK, Siegel NS. Sleep physiology, pathophysiology, and sleep hygiene. Prog Cardiovasc Dis. 2023 Mar-Apr;77:59-69. DOI: https://doi.org/10.1016/j.pcad.2023.02.005 Besedovsky L, Lange T, Born J. Sleep and immune function. Pflugers Arch. 2012 Jan;463(1):121-37. DOI: 10.1007/s00424-011-1044-0 Huang W, Ramsey KM, Marcheva B, Bass J. Circadian rhythms, sleep, and metabolism. J Clin Invest. 2011 Jun;121(6):2133-41. DOI: 10.1172/JCI46043 Korostovtseva L, Bochkarev M, Sviryaev Y. Sleep and Cardiovascular Risk. Sleep Med Clin. 2021 Sep;16(3):485-497. DOI: https://doi.org/10.1016/j.jsmc.2021.05.001 Lin J, Jiang Y, Wang G, Meng M, Zhu Q, Mei H, Liu S, Jiang F. Associations of short sleep duration with appetite-regulating hormones and adipokines: A systematic review and meta-analysis. Obes Rev. 2020 Nov;21(11):e13051. DOI: https://doi.org/10.1111/obr.13051 Liu S, Wang X, Zheng Q, Gao L, Sun Q. Sleep Deprivation and Central Appetite Regulation. Nutrients. 2022 Dec 7;14(24):5196. DOI: https://doi.org/10.3390/nu14245196 Morselli L, Leproult R, Balbo M, Spiegel K. Role of sleep duration in the regulation of glucose metabolism and appetite. Best Pract Res Clin Endocrinol Metab. 2010 Oct;24(5):687-702. DOI: https://doi.org/10.1016/j.beem.2010.07.005 Rogers EM, Banks NF, Jenkins NDM. The effects of sleep disruption on metabolism, hunger, and satiety, and the influence of psychosocial stress and exercise: A narrative review. Diabetes Metab Res Rev. 2024 Feb;40(2):e3667. DOI: https://doi.org/10.1002/dmrr.3667 Tobaldini E, Costantino G, Solbiati M, Cogliati C, Kara T, Nobili L, Montano N. Sleep, sleep deprivation, autonomic nervous system and cardiovascular diseases. Neurosci Biobehav Rev. 2017 Mar;74(Pt B):321-329. DOI: https://doi.org/10.1016/j.neubiorev.2016.07.004 Troynikov O, Watson CG, Nawaz N. Sleep environments and sleep physiology: A review. J Therm Biol. 2018 Dec;78:192-203. DOI: https://doi.org/10.1016/j.jtherbio.2018.09.012
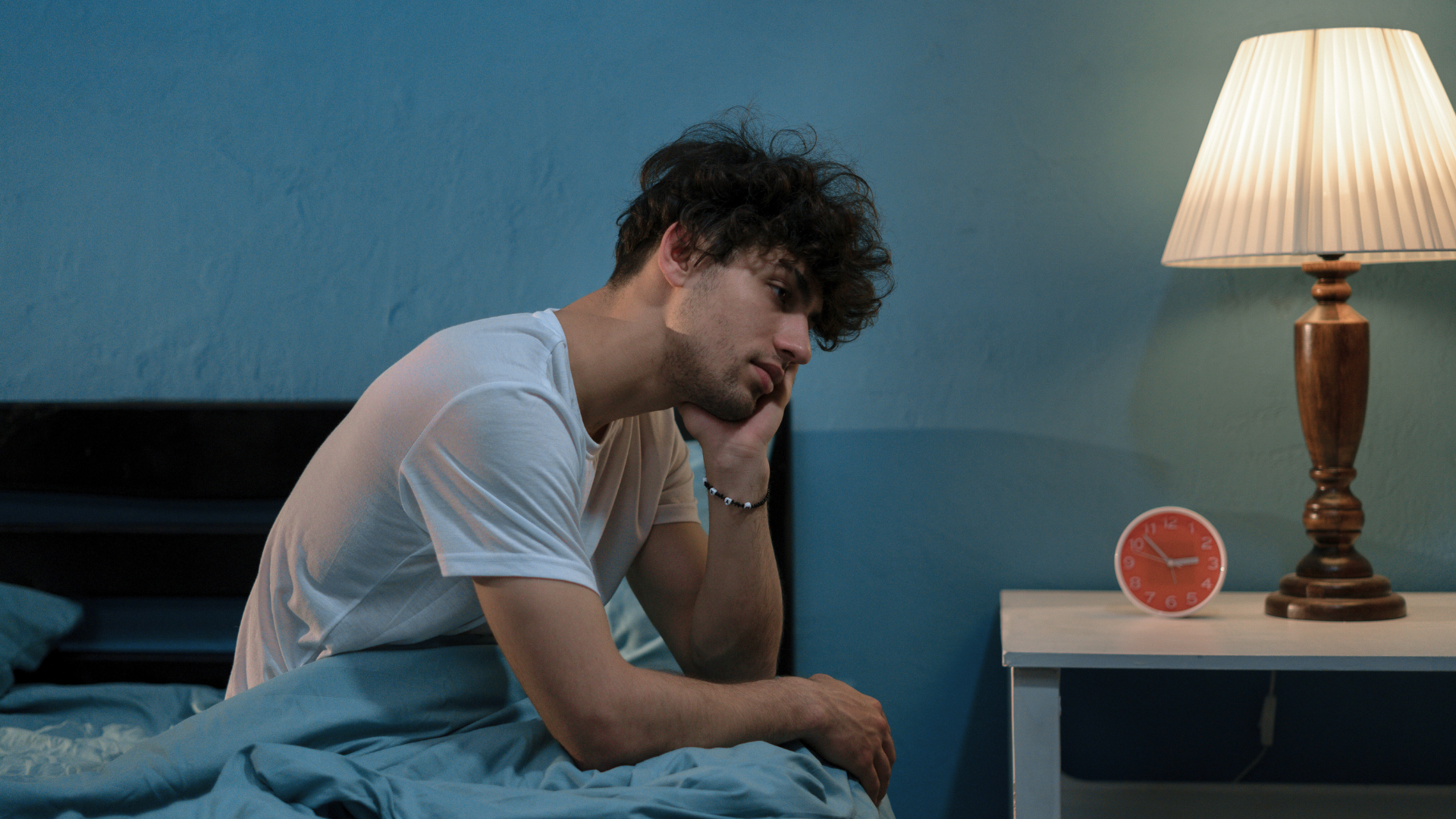
Key Points Healthy adults typically require a minimum of 7 hours of sleep per night, while sleep requirements are increased during infancy, childhood, and adolescence. Inconsistent sleep patterns are responsible for disrupting the circadian rhythm, promoting a vicious cycle of compromised sleep quality. Sleep consists of four vital stages: N1, N2, and N3, which constitute NREM (Non-Rapid Eye Movement) sleep, and a fourth stage of rapid eye movement (REM) sleep. During deep sleep, physiological processes such as the release of growth hormones occur, facilitating tissue recovery, muscle growth, and improving exercise performance It would not be surprising to acknowledge that getting enough sleep is challenging for many people. The fast-paced modern way of living has led to the sacrifice of ensuring the appropriate amount of sleep in order to cope with daily responsibilities, making sleep deprivation a global public health matter. Poor sleep, referring to both quantity and quality, is associated with numerous detrimental health outcomes, compromising cognitive, heart, and metabolic well-being. Yet, what defines poor sleeping status, and what is the ideal sleep regimen we should opt for? In the following article, there are going to be discussed the latest guidelines of sleep duration for all life stages, the different sleep stages and their importance regarding sleep quality, as well as the impact of sleep deprivation on recovery and exercise performance for all exercise modalities, including endurance, resistance, and HIIT training. Sleep duration guidelines and other factors of sleep quality Sleep duration recommendations vary across the lifespan. For healthy adults, the guidelines suggest 7 hours or more. Sleeping more than 9 hours appears to have substantial benefits only in younger adults or adults experiencing consistent sleep loss or illness. It is important to note that these recommendations do not apply to childhood, as children typically have higher sleep requirements to support growth and development. According to the National Sleep Foundation's latest guidelines, 14 to 17 hours is the goal set for newborns (0-3 months), 12 to 15 hours for infants, 9 to 12 hours for toddlers and preschoolers, and 8 to 10 hours for school-age children and adolescents. Alongside sufficient sleep duration, a healthy sleep state is also defined by high sleep quality, meaning the effective utilization of the time spent in bed for sleeping. Many exogenous factors can affect sleep quality, such as stress, anxiety, caffeine and alcohol intake, and inconsistent sleep schedules, leading to difficulties in sleep, insomnia, and frequent awakenings during the nighttime. Additionally, quality sleep suggests not only a regular sleep schedule but one that is also aligned with our internal clock-like regulation known as the circadian rhythm. The circadian rhythm regulates many aspects of the sleeping process and can be disrupted by many factors, including increased artificial light exposure and inconsistent sleep patterns. Hence, aligning our sleep schedule with our circadian rhythm is a principal way to ensure good quality sleep, as it ensures the optimal distribution of deep sleep and rapid eye movement (REM) sleep. The sleeping process consists of four stages: N1, N2, and N3, which constitute NREM (Non-Rapid Eye Movement) sleep, and a fourth stage of rapid eye movement (REM) sleep. NREM sleep reaches the deepest sleep state at stage N3, the most important stage of NREM sleep, determining sleep quality. However, experiencing REM sleep is also a significant indicator of high-quality sleep. REM is a critical phase of the sleep cycle that individuals experience in the latter stages of their sleep, preparing the brain to return to consciousness. During REM sleep, brain activity resembles that of wakefulness, making it a vital sleep phase for the brain to process feelings, sort out information, dream, and undergo restorative processes. Therefore, engaging in a healthy sleep regimen involves adherence to the recommended sleep duration guidelines according to age group as well as pursuing sleep quality in terms of optimal sleep timing and sleep stages duration. Sleep impact on recovery A robust body of evidence describes the restorative effect sleep exerts on the body's molecular and cellular processes, underlying the necessity of ensuring sufficient amounts of quality sleep. Sleep’s beneficial properties in cognitive function, immunity, and recovery from illness or injury have been attributed to the secretion of several growth hormones during different phases of the sleep period. As mentioned before, sleep involves four main stages. While all sleep stages are necessary components of the sleep cycle, the N3 stage plays a crucial role in recovery. Growth hormones, such as growth hormone and prolactin, which are vital for recovery, are highly released during this phase of deep sleep. Their secretion fosters injury healing by promoting tissue repair, regeneration, and muscle growth. Additionally, they exert beneficial properties by suppressing inflammation and promoting immunity strengthening. This enhances the body’s ability to address illnesses and infections efficiently. In addition, growth hormone release is associated with a significant decline in cortisol levels, the stress hormone. Cortisol typically exerts catabolic effects, and prolonged elevated cortisol levels are associated with increased tissue breakdown and immunity system suppression, thus delaying the process of healing and tissue repair. Cortisol levels, in alignment with the circadian rhythm, tend to peak during the day when the individual is awake and decrease at night as the body prepares for sleep. This decline in cortisol during nighttime allows growth hormones to facilitate tissue repair and healing effectively. It is also worth noting the action of another type of substances, cytokines. Cytokines are proinflammatory molecules released when an individual experiences a lack of sleep. Cytokines, like IL-6 and CRP, negatively affect the immunity system, impede muscle recovery, promote pain, and disrupt the autonomic nervous system balance that regulates our automatic bodily functions, such as heart rate, respiratory rate, and digestion. Therefore, their release in case of sleep deprivation can hinder efficient recovery and also exert detrimental effects on numerous vital organs. As a result, it’s clear that coordinated hormonal activity during sleep plays a crucial role in recovery. Therefore, prioritizing sleep quantity and quality that guarantees the experience of all necessary sleep stages is paramount for optimal recovery. Sleep impact on exercise performance Numerous studies have documented the critical role of sleep in optimizing exercise performance. Their findings underline that sleep, alongside training and nutrition, hold equivalent importance as fundamental factors for peak performance. For professional athletes, sleep requirements increase to 9 to 10 hours/day due to the increased physical and mental demands of their training sessions. While extended sleep duration is needed to ensure proper physical and cognitive recovery, most athletes find it difficult to attain sufficient sleep. This challenge stems from busy schedules, irregular sleep patterns, time-zone changes during competition periods, and the anxiety and stress they face, leading to severe sleep restriction or deprivation. According to published data, sleep restriction or deprivation that both athletes and exercising individuals often experience seriously impacts athletic performance. This is associated with diminished ability to reach peak performance, injury susceptibility, and delayed recovery, emphasizing the significance of preserving optimal sleep patterns. Endurance exercise Low-to-moderate intensity endurance exercise that reaches 50-75% of an individual’s VO2max, such as running, rowing, cycling, etc., demands high cardiorespiratory activity and sustained effort for extended training periods. Sleep, alongside other critical factors, plays a fundamental role in achieving peak performance in this type of exercise as it contributes to efficient energy replenishment. Sleep, mainly NREM sleep, achieves that by facilitating glycogen synthesis and storage in muscles and the liver, which is a primary energy resource during endurance exercise. Moreover, growth hormone that is extensively secreted during this sleep phase promotes muscle recovery and the storage of glucose as glycogen, contributing to efficient energy replenishment. Therefore, sleep restriction or deprivation contributes to decreased energy fuels and impaired muscle repair, ultimately diminishing the ability to maintain sustained effort during endurance activities. Sleep deprivation has also significant impacts on cognitive function and mood. Many studies have demonstrated decreased strength, stamina, and motivation after overnight sleep loss or reduced sleep duration, leading to decreased athletic performance. Sleep deprivation also appears to increase the perception of the endurance athletes' effort, contributing to greater fatigue and exhaustion. This can elevate the risk of injuries as athletes push themselves trying to overcome the perceived strains. Lastly, feelings of frustration, anger, and confusion can also result from insufficient sleep, especially REM sleep, which is vital for cognitive restoration. Resistance training Resistance training imposes significant strain on the muscles, making sufficient sleep a priority to attain peak performance. As mentioned before, hormones are released during the nighttime, inducing muscle repair and growth, an essential metabolic procedure for gaining strength. While research data on the impact of sleep deprivation on resistance training performance is inconclusive, insufficient muscle repair and recovery seems to be the most likely underlying factor contributing to potential declines in performance. In terms of cognitive function, sleep deprivation can alter the athletes’ psychomotor skills and affect their coordination, reaction time, and ability to make decisions, all parameters necessary for peak performance during resistance training. High-Intensity Interval Training (HIIT) Sleep is crucial in high-intensity interval training performance as well. Specifically, getting the adequate amount of sleep enhances the body’s ability to meet the increased HIIT demands by improving stamina and promoting muscle recovery through nighttime glycogen synthesis and hormonal regulation. Similar to resistance training, HIIT requires proper cognitive function. Mental focus, coordination, and rapid reaction times are some of the parameters of cognitive function required during HIIT training, which appear to be threatened by sleep deprivation. To sum up, sleep is frequently overlooked yet fundamentally crucial for overall health, with both quantity and quality holding great significance. Quality sleep assists in preserving good health by ensuring the accomplishment of the necessary sleeping stages and the optimal secretion of hormones responsible for growth and repair. Moreover, besides recovery and tissue repair, adequate sleep is crucial for performance optimization by enhancing physiological and cognitive performance abilities. REFERENCES Barbato G. REM Sleep: An Unknown Indicator of Sleep Quality. Int J Environ Res Public Health. 2021 Dec 9;18(24):12976. DOI: https://doi.org/10.3390/ijerph182412976 Charest J, Grandner MA. Sleep and Athletic Performance: Impacts on Physical Performance, Mental Performance, Injury Risk and Recovery, and Mental Health. Sleep Med Clin. 2020 Mar;15(1):41-57. DOI: https://doi.org/10.1016/j.jsmc.2019.11.005 Doherty R, Madigan SM, Nevill A, Warrington G, Ellis JG. The Sleep and Recovery Practices of Athletes. Nutrients. 2021 Apr 17;13(4):1330. DOI: 10.3390/nu13041330 Fullagar HH, Skorski S, Duffield R, Hammes D, Coutts AJ, Meyer T. Sleep and athletic performance: the effects of sleep loss on exercise performance, and physiological and cognitive responses to exercise. Sports Med. 2015 Feb;45(2):161-86. DOI: https://doi.org/10.1007/s40279-014-0260-0 Hirshkowitz M, Whiton K, Albert SM, Alessi C, Bruni O, DonCarlos L, Hazen N, Herman J, Adams Hillard PJ, Katz ES, Kheirandish-Gozal L, Neubauer DN, O'Donnell AE, Ohayon M, Peever J, Rawding R, Sachdeva RC, Setters B, Vitiello MV, Ware JC. National Sleep Foundation's updated sleep duration recommendations: final report. Sleep Health. 2015 Dec;1(4):233-243. DOI: https://doi.org/10.1016/j.sleh.2015.10.004 Vitale KC, Owens R, Hopkins SR, Malhotra A. Sleep Hygiene for Optimizing Recovery in Athletes: Review and Recommendations. Int J Sports Med. 2019 Aug;40(8):535-543. DOI: 10.1055/a-0905-3103 Watson AM. Sleep and Athletic Performance. Curr Sports Med Rep. 2017 Nov/Dec;16(6):413-418. DOI: 10.1249/JSR.0000000000000418 Watson NF, Badr MS, Belenky G, Bliwise DL, Buxton OM, Buysse D, Dinges DF, Gangwisch J, Grandner MA, Kushida C, Malhotra RK, Martin JL, Patel SR, Quan SF, Tasali E. Recommended Amount of Sleep for a Healthy Adult: A Joint Consensus Statement of the American Academy of Sleep Medicine and Sleep Research Society. Sleep. 2015 Jun 1;38(6):843-4. DOI: 10.5665/sleep.4716
Ready to take control of your health?
Book a free consultation.